Overwork-related deaths (karoshi) or disorders are major occupational issues, and related studies have been conducted not only in Japan (1), but also in other countries (2). In Japan, the Ministry of Health, Labour and Welfare has reported the “Status of Industrial Accident Compensation for Karoshi, etc.”, which is the annual number of compensated occupational cerebrovascular and cardiovascular diseases and mental disorders since 1988 (3). The annual numbers of compensated cases of occupational cerebrovascular and cardiovascular diseases for 2012–2016 were 338, 306, 277, 251, and 260, respectively (3). However, the decline is minimal suggesting that the issues remain unresolved. To prevent the occurrence of health disorders associated with overwork, studies examining the health effects of overwork are important and urgently required.
One of the factors inducing several negative effects on workers’ cardiovascular health is long working hours (LWH). Some systematic reviews that conducted meta-analysis for coronary heart disease (4, 5) and stroke (4) reported that LWH groups were associated with an increased risk of coronary heart disease and stroke compared to control groups without LWH. In Japan, the Labour Force Survey (6) conducted by the Statistics Bureau, Ministry of Internal Affairs and Communications reported that 4.33 million workers (7.8%) worked >60 hours/week in 2016. These studies suggested that LWH enhances the cardiovascular burden and increases cardiovascular diseases’ risk, while a lot of workers suffer due to LWH. On the other hand, several cohort studies reported that LWH was associated with increased blood pressure (BP) among assembly-line workers, but not clerks and engineers/special technicians (7) and with an increased risk of hypertension (8). However, another cohort study (9) reported that LWH was associated with decreased BP and risk of hypertension. Other previous studies which examined the acute effects of LWH also showed conflicting results. For example, one study reported that LWH increased 24-hour ambulatory BP (10), but another study reported that LWH did not elevate ambulatory BP (11). The inconsistency in the results from previous studies suggests that factors other than LWH that influenced BP may exist. Previous studies have reported that there are many factors which influence cardiovascular responses in the real workplace such as occupational stress (12), social support (13), and occupational noise (14, 15). To minimize the influences of these occupational factors, it is important to examine cardiovascular responses under LWH in a controlled laboratory environment.
On the other hand, it was also reported that hypertension was associated with cardiovascular health. A meta-analysis (16) of 61 prospective observational studies (958 074 participants, 40–89 years old) examined the relationship between BP and vascular mortality. Findings showed that systolic BP (SBP) and diastolic BP (DBP) are strongly and directly associated with vascular mortality. In these studies, individuals with high BP showed higher risk of vascular mortality. The National Health and Nutrition Survey (17) conducted by the Japanese Ministry of Health, Labour and Welfare reported that 46.9% (N=3539) individuals had hypertension (over 20 years, the survey included non-workers) in 2015. These studies suggest that hypertension increases the risk of cardiovascular diseases and that a lot of the workers have hypertension.
Consequently, it may be thought that a lot of workers with hypertension are working under LWH and suffer more severe cardiovascular burden through LWH compared with normotensive workers. Hayashi et al (10) measured the 24-hour BP of male white-collar workers with (SBP 140–160 mmHg or DBP 90–105 mmHg) and without (SBP <140 mmHg and DBP <85 mmHg) hypertension, and reported that BP was higher among workers with long overtime work (≥60 hours/month) than those with short overtime work (≤30 hours/month) in both groups. Conversely, this study did not compare the BP between hypertensive and normotensive workers. In addition, the changes that occurred in the underlying hemodynamic responses with increasing BP as a result of LWH and the differences in the variation between the hypertensive and normotensive groups were not clear. Moreover, as described above, many factors (eg, social support (13)) have been reported to impact cardiovascular responses. Therefore, it is important to examine the influences of LWH on hemodynamic responses under a controlled laboratory environment to reduce bias due to confounding factors.
The present experimental study compared hemodynamic responses due to simulated LWH (13 hours) between participants suspected of having hypertension and normotensive participants in the laboratory. We tested the following two hypotheses. Hypothesis 1: hemodynamic responses will gradually deteriorate with work time extension. Hypothesis 2: deterioration of hemodynamic responses will be more noticeable among the participants suspected of having hypertension than normotensive participants.
Methods
Participants
Participants were recruited through a temporary-employment agency, which has a database of potential participants who registered as subjects beforehand. The recruitment information was shown in a website for the current study and e-mails were also sent to potential participants. The website indicated inclusion criteria to participate in this study: age (30–59 years), gender (men only), health status in general (individuals had no previous history of cardiac disease, diabetes, asthma, cerebral stroke, chronic liver disorder, back problems, and mental disorders), and BP category [normotensive (resting SBP ≤140 mmHg, and resting DBP ≤90 mmHg) or grade 1 hypertension (resting SBP=140-159 mmHg or DBP=90–99 mmHg)]. The potential participants could then access the website and register for an interview. Resting BP of all potential participants was confirmed on the interview day, and those with BP higher than the recruitment range (SBP >160 mmHg or DBP >100 mmHg) were excluded. As a result of the interview, 35 men were recruited for this study. They were categorized into a normotensive group [SBP ≤140 mmHg and DBP ≤90 mmHg of resting BP, N=22; mean age=49.0 years; standard error of the mean (SEM)=1.0] and a high BP group (SBP=140–160 mmHg or DBP=90–100 mmHg of resting BP, N=13; mean age=51.9 years; SEM=1.5). None of the participants used antihypertensive drugs. The experimenter (nurse) interviewed all participants. They were asked to abstain from substances that can affect the experiments (such as alcohol, caffeine, and nicotine) during the experimental period, and asked to sleep >6 hours before the experimental day.
All participants gave written informed consent before the experiment. The Research Ethics Committee of the National Institute of Occupational Safety and Health, Japan, approved the study (H2713).
Procedures
The experiment spanned two inconsecutive days. On the first day, participants were informed of the experimental protocol and practiced all the experimental tasks; additionally, participants’ characteristics (height, weight, smoking status, and resting BP) were measured. The first day started at 14:00 or 14:30 hours and the procedures were carried out for approximately two hours. Resting BP (SBP and DBP) was measured twice using the arm-cuff digital BP monitor (CH-463E; Citizen Systems Japan Co, Ltd, Tokyo, Japan), and the mean values were used to categorize the groups.
The second day was the experimental day. Participants came to the laboratory at approximately 08:30 hours. Figure 1 shows the experimentally simulated LWH protocol. First, the participants’ hemodynamic responses were measured at baseline (resting condition in a sitting position) from 09:00–09:10 hours. Then, they conducted the simulated LWH from 09:10–22:00 hours (almost 13 hours of work). Simulated LWH consisted of 12 mental work task sessions (45 minutes) and breaks (10–60 minutes) between sessions. Hemodynamic responses were measured in a sitting position under the working conditions during the last 5 minutes of the task sessions. Hemodynamic responses measured at baseline and during the simulated LWH included the SBP, DBP, mean arterial pressure (MAP), stroke volume (SV), heart rate (HR), cardiac output (CO), and the total peripheral resistance (TPR). These were measured continuously during 5 minutes from a middle finger by the non-invasive monitor (Finometer pro; Finapres medical systems, Amsterdam, The Netherlands).
Tasks
In each session, one of three mental work tasks (color word task, mental arithmetic task, and number copy task) was conducted. We combined the three sessions into one block, each task was conducted only once in a block. Task order among blocks and participants was randomized.
The color word task presented a target word, which was the name of a color (for example, red), that was printed in a different color from the meaning of the word (for example, green). Around the target word, six buttons labelled with the names of colors (black, red, yellow, blue, green, and purple) were presented. The participants were instructed to press the button corresponding to the color of the target word within 10 seconds. The mental arithmetic task presented two random 2-digit numbers (range 10–49) on the computer screen. Participants were asked to add them up mentally and type the result within 20 seconds using a 10-key pad. The number copy task presented a random 10-digit number on a computer screen. Participants were asked to type the same number within 20 seconds using a 10-key pad. All tasks were repeated during the task sessions (45 minutes).
Analysis
Unpaired t-tests were used to examine between-group differences in BP on the first day, and in age, body mass index (BMI), and baseline measurements (SBP, DBP, MAP, SV, HR, CO, and TPR) on the second day. A chi-squared test was conducted to examine the group differences in smoking status. Two-way repeated ANOVAs [groups (2) × blocks (4)] were conducted for correct response rate and reaction time for each task (color word task, mental arithmetic task, and number copy task) to explore the group differences in task performance. To explore the influences of LWH on hemodynamic responses of the normotensive and high BP groups, the different values of the hemodynamic responses were calculated by subtracting each session’s value from the baseline value; two-way repeated ANOVAs [groups (2) × sessions (12)] were then conducted based on the different values of the hemodynamic responses (ΔSBP, ΔDBP, ΔMBP, ΔSV, ΔHR, ΔCO, and ΔTPR). Hypotheses 1 and 2 were tested by the main effect of the session and interaction (group × session) respectively. Degrees of freedom were adjusted via the Greenhouse-Geisser epsilon calculation. All ANOVAs were followed by post-hoc tests with Bonferroni corrections, and a post-hoc test of sessions was conducted to compare session 1 with the other sessions.
All statistical analyses were conducted using SPSS version 23.0 for Microsoft Window (IBM Corp, Armonk, NY, USA).
Results
Hemodynamic responses were missing for one participant in the normotensive group due to technical problems. The data of this participant was excluded from subsequent analyses. Table 1 shows the characteristics of the two groups. Unpaired t-tests revealed that although SBP (t=6.46, df=32, P<0.001) and DBP (t=7.16, df=32, P<0.001) were significantly higher in the high BP group than in the normotensive group on the first day, there were non-significant (NS) differences in age and BMI (all P>0.10). A chi-squared test revealed that there was no significant difference in smoking status between the groups (χ2=0.35, df=2, NS). Additionally, at baseline, the results of hemodynamic responses on the second day, SBP (t=3.95, df=32, P<0.001), DBP (t=2.72, df=32, P<0.05), and MAP (t=4.02, df=32, P<0.001) were significantly higher in the high BP group than the normotensive group, but there was no significant difference in SV, HR, CO, and TPR between the groups (all P>0.10). Table 2 shows the results for the mental arithmetic, color word, and number copy tasks. A two-way repeated ANOVA (group × block) revealed no significant main or interaction effects for the reaction times or the correct response rates.
Table 1
Characteristics of the groups [SEM=standard error of the mean; MU=medical unit; NS=non-significant; BP=blood pressure].
Variables | Normotensive group (N=21) | High blood pressure group (N=13) | P-value | ||||||
---|---|---|---|---|---|---|---|---|---|
|
|
||||||||
Mean | SEM | N | % | Mean | SEM | N | % | ||
Age (years) | 49.19 | 1.00 | 51.85 | 1.51 | NS a | ||||
Body mass index | 23.98 | 0.69 | 22.88 | 0.67 | NS a | ||||
Smoking status | |||||||||
Non-smoker | 12 | 57 | 7 | 32 | NS b | ||||
Ex-smoker | 6 | 29 | 4 | 18 | |||||
Current smoker | 3 | 14 | 2 | 9 | |||||
BP on 1st day c | |||||||||
Systolic (mmHg) | 118.26 | 2.08 | 139.77 | 2.57 | <0.001 a | ||||
Diastolic (mmHg) | 79.07 | 1.54 | 95.12 | 1.36 | <0.001 a | ||||
Hemodynamic responses on 2nd day: baseline d | |||||||||
Systolic BP (mmHg) | 117.46 | 1.84 | 131.72 | 3.46 | <0.001 a | ||||
Diastolic BP (mmHg) | 72.26 | 1.17 | 79.29 | 2.72 | <0.05 a | ||||
Mean arterial pressure (mmHg) | 89.85 | 1.32 | 101.11 | 2.87 | <0.001 a | ||||
Cardiac output (l/min) | 5.51 | 0.25 | 5.63 | 0.33 | NS a | ||||
Heart rate (bpm) | 71.54 | 2.66 | 75.39 | 2.98 | NS a | ||||
Stroke volume (ml) | 77.64 | 2.57 | 75.39 | 4.23 | NS a | ||||
Total peripheral resistance (MU) | 1.03 | 0.05 | 1.16 | 0.10 | NS a |
Table 2
Reaction time and correct response rate for each block of each task [SEM=standard error of the mean; ms=milliseconds].
Figure 2 shows the mean difference of the values for ΔSBP, ΔDBP, ΔMAP, ΔSV, ΔCO, ΔHR, and ΔTPR in both groups at each session. The two-way repeated ANOVA for the ΔSBP showed a significant interaction [F(5.12, 163.70)=3.03, P<0.05, partial η2=0.086]. Analysis of the simple main effects revealed that there were significant main effects of the session in both normotensive [F(11, 352)=4.16, P<0.001] and high BP [F(11, 352)=13.11, P<0.001] groups. Table 3 shows the results of the post-hoc tests of the interaction or main effects of the session on hemodynamic responses. Post-hoc tests in the normotensive group revealed that the ΔSBP were higher in the sessions 7–10, and session 12 than session 1 (all P<0.05). Post-hoc tests in the high BP group revealed that the ΔSBP was higher in sessions 7–12 than session 1 (all P<0.05). Additionally, analysis of the simple main effects revealed that the ΔSBP was significantly higher in the high BP group than the normotensive group in session 6 [F(1,32)=5.26, P<0.05], session 8 [F(1,32)=9.74, P<0.01], session 9 [F(1,32)=15.67, P<0.001], session 11 [F(1,32)=4.76, P<0.05], session 12 [F(1,32)=9.10, P<0.01].
Figure 2
Mean difference values (value at session – values at baseline) for systolic blood pressure, diastolic blood pressure, mean arterial pressure, cardiac output, stroke volume, heart rate, and total peripheral resistance in each session. Error bars indicates the standard error of the mean (SEM). * indicates significant differences (P<0.05) between the normotensive group and the high blood pressure group.
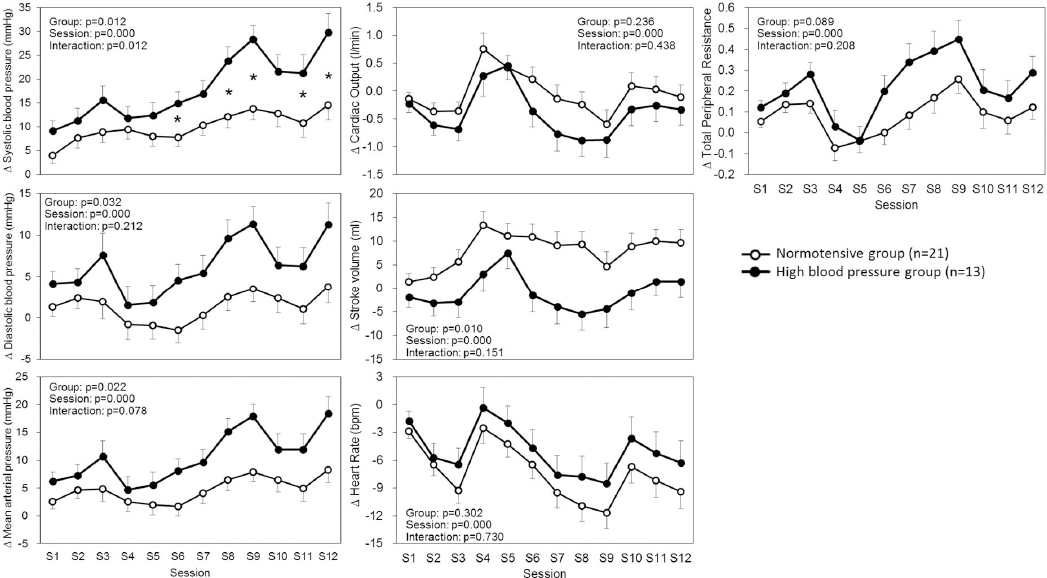
Table 3
The results of the post-hoc tests of interactions (systolic blood pressure) and main effect of sessions (other hemodynamic responses) compared with session 1.
Significant differences compared with session 1 (S1) | |||||||||||
---|---|---|---|---|---|---|---|---|---|---|---|
|
|||||||||||
S2 | S3 | S4 | S5 | S6 | S7 | S8 | S9 | S10 | S11 | S12 | |
ΔSystolic blood pressure a | |||||||||||
Normotensive group | 1 b | 1 b | 1 b | 1 b | 1 b | ||||||
High blood pressure group | 1 b | 1 b | 1 b | 1 b | 1 b | 1 b | |||||
ΔDiastolic blood pressure c | 1 b | ||||||||||
ΔMean arterial pressure c | 1 b | 1 b | 1 b | ||||||||
ΔCardiac output c | 1 b | ||||||||||
ΔStroke volume c | 1 b | 1 b | |||||||||
ΔHeart rate c | 2 d | 2 d | 2 d | 2 d | 2 d | 2 d | |||||
ΔTotal peripheral resistance c | 1 b |
The ANOVA for the ΔDBP revealed a significant main effect of group [F(1.00, 32.00)=5.06, P<0.05, partial η2=0.137] and session [F(5.57, 178.15)=7.74, P<0.001, partial η2=0.195], but a non-significant interaction [F(5.57, 178.15)=1.42, NS]. Post-hoc tests of sessions showed that the ΔDBP was higher in session 9 than in session 1 (P<0.05). The ANOVA for the ΔMAP revealed a significant main effect of group [F(1.00, 32.00)=5.82, P<0.05, partial η2=0.154] and session [F(5.41, 173.21)=12.46, P<0.001, partial η2=0.280], but a non-significant interaction [F(5.41, 173.21)=1.98, P<0.10]. Post-hoc tests of the sessions showed that the ΔMAP was higher in sessions 8, 9, and 12 than session 1 (all P<0.05).
The ANOVA for the ΔCO revealed a significant main effect of sessions [F(5.07, 162.21)=12.99, P<0.001, partial η2=0.289], but no significant main effect of group [F(1.00, 32.00)=1.46, NS] or interaction [F(5.07, 162.21)=0.97, NS]. Post-hoc tests of session showed that the ΔCO was higher in session 5 than session 1 (P<0.05). The ANOVA for the ΔSV revealed a significant main effect of group [F(1.00, 32.00)=7.44, P<0.05, partial η2=0.189] and session [F(5.71, 182.86)=5.41, P<0.001, partial η2=0.145], but a non-significant interaction [F(5.71, 182.86)=1.61, NS]. Post-hoc tests of session showed that the ΔSV was higher in sessions 4 and 5 than in session 1 (all P<0.05). The ANOVA for the ΔHR revealed a significant main effect of session [F(3.41, 109.24)=20.57, P<0.001, partial η2=0.391], but no significant main effect of group [F(1.00, 32.00)=1.10, NS] or interaction [F(3.41, 109.24)=0.47, NS]. Post-hoc tests of session showed that the ΔHR was lower in sessions 2, 3, 7–9, and 12 than session 1 (all P<0.05).
The ANOVA for the ΔTPR revealed a significant main effect of session [F(5.18, 165.64)=13.51, P<0.001, partial η2=0.297], but no significant main effect of group [F(1.00, 32.00)=3.07, P<0.10] or interaction [F(5.18, 165.64)=1.45, NS]. Post-hoc tests of session showed that the ΔTPR was higher in session 9 than session 1 (P<0.05).
Discussion
In the present study, although the ΔSBP, ΔDBP, ΔMAP, ΔHR, and ΔTPR were higher in later sessions of work than the initial session (session 1), the ΔCO and ΔSV were not, suggesting partial support for hypothesis 1. Furthermore, only the ΔSBP in later sessions were higher in the high BP group than in the normotensive group, and the result is partially consistent with hypothesis 2. These results showed that LWH increased BP, with a larger increase in SBP among individuals suspected of having hypertension.
A possible explanation for the increased SBP in the high BP group due to LWH is the excessive increase and delayed recovery of BP under stressful conditions among hypertensives. A previous study (18) reported that BP in hypertensives showed greater activation and slower recovery from stress compared with the normotensives. Increased BP responses accumulated due to LWH stress and resulted in a higher ΔSBP in the high BP group during later sessions. Previous studies also reported that not only LWH (4, 5) but also hypertension (16, 19) increased the risk of cardiovascular diseases. Furthermore, it was reported that a greater increase in cardiovascular response to mental stress was associated with poor subsequent cardiovascular status which includes cardiovascular disease events (20). These results suggest that hypertensive workers with LWH may have an increased risk of cardiovascular disease. In Japan, it is thought that a lot of workers with hypertension are subject to LWH (6, 17). Therefore, workers, especially those with hypertension, should implement preventive measures to reduce cardiovascular burden due to LWH. Such preventive measures include the avoidance of LWH, management of BP, and ensuring adequate rest time for recovery from fatigue.
A previous study (10) reported that the 24-hour ambulatory average BP of normotensive and hypertensive workers who worked overtime work was higher than in workers who did not work overtime. The present study supports the findings that increased BP during the overtime working hours may increase the 24-hour average BP. On the other hand, it is possible that LWH over many days may result in increased BP in the early hours. Further studies are therefore needed to clarify the effects of LWH over many days on workers cardiovascular health.
The present study also measured the underlying hemodynamic responses that increase BP, such as cardiac responses (CO, HR, and SV) and vascular responses (TPR) during LWH. Although SV at baseline did not differ between the groups, the ΔSV during LWH was lower in the high BP group than in the normotensive group. The low ΔSV in the high BP group may be due to the deterioration of cardiac function since SV is an index of the cardiac response. Conversely, other cardiac responses (CO and HR) did not differ between groups. The cardiac and vascular responses corresponding to BP elevation due to LWH were not significant. Therefore, the underlying hemodynamics which causes an excessive increase in BP in participants suspected of having hypertension is unclear. It is known that hemodynamic responses are complicated and patterns of the cardiac and vascular responses to mental work were dramatically different among individuals. Previous studies (21–23) reported that there were three patterns of hemodynamic responses to mental tasks: the cardiac pattern which increases CO and decreases TPR, the vascular pattern which increases TPR and decreases CO, and the mixed pattern which moderately increases both CO and TPR. It is possible that the underlying hemodynamics, which causes an excessive increase in BP in participants suspected of having hypertension, could be clarified by further analysis of the sub-group hemodynamic response patterns. However, the present study could not be used to analyze such sub-groups due to the small sample size of the high BP group. Further studies with larger sample sizes of individuals with high BP group are needed to clarify this issue.
Although there were main effects of session in all hemodynamic responses, only the ΔSBP, ΔMAP, and ΔHR were significantly changed at end of LWH (session 12) compared to the start of LWH (session 1). These results suggest that hypothesis 1 was partially supported. The unpredictable variations over simulated LWH on other hemodynamic responses may have been caused by the two breaks between sessions 3 and 4 (60-minute rest) as well as between sessions 9 and 10 (50-minute rest). When the data of these sessions were excluded, the variation of sessions for all hemodynamic responses was practically the same. These breaks allowed the participants to get away from the tasks, and although the sensitivity to breaks may be different for individual outcomes, lack of accumulation of burden due to work and recovery from the burden may occur and affect hemodynamic responses.
BP has a circadian rhythm, usually causing an increase in BP in the early morning hours, high levels during the daytime with peak values between mid-morning and noon, falling progressively throughout the day, and dipping during the night (24, 25). Although, this pattern did not differ between normotensives and hypertensives, BP in hypertension was higher than in normotensives (24, 25). In the present study, BP increased further during the later sessions (overtime working hours) in both groups; this increased BP in the later sessions may be due to the LWH and not circadian rhythms. On the other hand, the circadian rhythms of BP could be masked by work activity (26). In the present study, there was no control group that finished mental tasks after session 8 (normal 8 hours labor). Therefore, we are not sure whether further rise of BP after 8 hours of work (session 9-12) is different from the pattern of BP after a normal 8-hour work shift. Additional studies that control for this condition in the control group are needed to clarify the issue.
The present study had several limitations. First, a statistical power analysis was not conducted to determine the sample size, and the sample size used here may have been small. This potential weakness can produce unreliable results. Second, although the SBP and DBP in usual (before experiment) and on the first day of the study were higher than clinical levels of hypertension (SBP=140–160 mmHg or DBP=90–100 mmHg of resting BP), the SBP and DBP on the second day were below clinical levels. This discrepancy is likely due to differences in instructions provided regarding participants behaviors which may affect BP, including getting >6 hours of sleep and abstaining from caffeine, alcohol and nicotine. Such instructions were provided on day 2 but not on day 1. The instructions and controlled behaviors only on day 2 may have decreased BP in the high BP group. Additionally, the time of day at which BP measurements were taken distinctly differed between day 1 (ranged from 14:00–16:30 hours) and the baseline on day 2 (09:00–09:10 hours). As previously indicated, BP has a circadian rhythm, and the time difference may have caused the difference in BP between day 1 and the baseline on day 2. Additional studies that control for these conditions are needed. Third, the participants in this study were >40 years of age. Thus, the effects of LWH on young workers (<40 years of age) are not clear. Fourth, this study recruited only men since it was reported that the menstrual cycle affects cardiovascular responses to stress (27). Thus, it is not clear whether there are gender effects on the relationship between LWH and hemodynamic responses. Fifth, several factors which influence hemodynamic responses were controlled under the laboratory environment in the present study, but these factors may occur under the LWH in real working places. Additionally, some conditions, for example the work content, may differ between the present study and real office work. Therefore, the changes in BP due to LWH may be enhanced or reduced in some working places. Further field studies are therefore needed. Sixth, although the present study revealed the effects of LWH on hemodynamic responses while performing mental tasks, the effects of LWH while performing physical tasks are not clear. Further studies are needed to clarify the effects of LWH with physical work. Seventh, a previous study has reported that increased effort causes higher BP (28). This might explain our current findings; however we are unable to test this possibility since the efforts exerted by the participants during the tasks were not measured. Therefore it is not clear whether there was a group difference in effort. Finally, it is not clear how the experimental findings in the present study are linked to the actual cardiovascular events.
In conclusion, our study found that LWH increases BP, with a larger increase in SBP in the later working hours among individuals with untreated hypertension, whereas other hemodynamic responses did not differ between groups as a function of the LWH. Although there are limitations mentioned above, these findings provide an improved understanding of the changes that occur in hemodynamic responses with simulated LWH while performing mental tasks. Thus they may contribute to the body of evidence on the relationship between LWH and hypertension.