Workers in the aluminum industry are exposed to particulate matter at each step of the manufacturing processes, from mining to refining, smelting and fabricating. Exposure to particles and associated potential adverse health effects in this industry have been evaluated previously (1–4). Although respirators have been known to be used in the industry, the pattern of the use and its impact on workers’ exposure, including exposure to particulate matter, have not been reported in published literature. During an epidemiologic study, evaluating fine particulate matter exposure and risk of cardiovascular disease (5–9), we obtained industrial hygiene measurement data collected over past decades at >200 facilities of a major US aluminum manufacturer. Respirators were found to be used often in these facilities especially since late 1980s. This manuscript describes the pattern of respirator use in 12 facilities that were included in the epidemiologic study, with emphasis on exposure to total particulate matter (TPM). The impact of respirator use on estimated TPM exposure was evaluated. Respirator-use data and analysis results reported here will be useful for industrial hygiene professionals in general and occupational health researchers conducting health studies of particulate matter exposure in the industry.
Methods
The electronic database contained industrial hygiene sampling data from 1966–2013, including chemical/physical agents, sampling date, facility, department, job, task, sample type (personal/area, full/partial shift and routine/diagnostic), shift (day/evening/night), sampling duration and personal protective equipment used by the employee who wore the sampling device. Descriptive analyses were restricted to full-shift personal TPM samples collected in 12 facilities that were included in the epidemiologic study to evaluate respirator use over time among the facilities, by facility type and by job. The impact of respirator use on TPM exposure was evaluated by examining the number jobs affected by the respirator-use adjustment and the reduction in estimated TPM exposure due to the adjustment for those jobs. Our determination of respirator use was based on two variables in the database: “respirator_style” and “respirator_type.” If a respirator was observed to be used by the worker who wore the TPM sampler, respirator style and type were recorded for that TPM measurement. Respirator style included full facepiece, half mask, quart mask and helmet/hood. Whereas recorded respirator type were air-purifying respirator, powered air-purifying respirator (PAPR), supplied-air respirator (SAR) and self-contained breathing apparatus (SCBA) (table 1). Respirator use was adjusted by both assigned protection factors (APF) and workplace protection factors (WPF).
Table 1
Assigned protection factors (APF) and workplace protection factors (WPF) use in this study.
Assigned protection factor
We used APF listed in the US Code of Federal Regulations (29 CFR) Occupational Safety and Health Administration (OSHA) Respiratory Protection Program (10). To be conservative, we assumed SAR and SCBA were operated under demand mode as information on the operation mode was not provided in the database (APF=10, 50 for SAR half mask and full facepiece, respectively, and APF=50 for SCBA full facepiece) (table 1). We followed OSHA technical notes that stated all PAPR and SAR with helmets/hoods should be treated as loose-fitting facepiece respirators (APF=25), unless the employer provided evidence from the respirator manufacturer that demonstrated the performance at a level of protection of ≥1000.
Workplace protection factor
WPF were determined by either directly adopting APF or applying a safety factor to APF. Nicas & Neuhaus (11) investigated data from seven studies of negative-pressure air-purifying half-mask respirators and analyzed within- and between-wearer variance components. They found substantial variability in WPF within-wearer from wearing to wearing, as well as between wearers. Therefore, they recommended reducing the current half-masking APF from 10 to 5 to properly account for those variations. Based on this analysis, we decided to use half of current APF as WPF for negative-pressure air-purifying respirators, including full facepieces, half masks and quarter masks (table 1). We also used half of current APF as WPF for respirators operated under demand mode (half mask SAR, full facepiece SAR/SCBA and helmet/hood SCBA) because of possible negative pressure in the facepiece under the demand mode. We used APF as WPF for respirators operate under positive pressure, including full face PAPR and helmet/hood with powered air or supplied air.
These APF and WPF were applied to individual TPM measurements where respirator use was recorded for the samples, based on the type and style of respirator recorded in the database. TPM exposure for a given job (unadjusted and adjusted) was estimated by the job mean TPM concentration, which was calculated by averaging all TPM measurements (unadjusted and adjusted, respectively) collected for the job over time. The number of job affected by the respirator use and the magnitude of reduction in the job mean TPM concentration were evaluated to examine the impact of respirator use on TPM exposure. Finally, job exposure matrices (JEM) containing both unadjusted and adjusted TPM (APF and WPF) exposure estimates were developed (supplementary table S1, www.sjweh.fi/show_abstract.php?abstract_id=3735). Given that respirator use changed substantially over time, the JEM included temporal trends by presenting job mean TPM estimates in three time periods, before 1990, 1990–1999, and after 1999. Details on the approach to the development of the JEM have been described previously (6).
Results
Respirator use over time
figure 1 illustrates the pattern of respirator use among full-shift personal TPM measurements (N=12 402) at 12 facilities over time. These facilities included a refinery (facility A), 5 smelters (facilities B, C, D, E and F) and 6 fabricating facilities (facilities G, H, I J, K and L). Of the smelters, 2 (B and C) contained fabricating units. Some facilities had less data available in the database compared to others. For example, 4 factories were acquired by the company in late 1990s and early 2000s and thus had much less data (facility F in 1999, facilities J, K and L in 2002). Electronic data were available for facility C only after 1991. Records for other facilities went back to 1980s in the database. Among these 12 plants, first recorded respirator use appeared in facility B in 1990, followed by facilities C and D in 1991, facilities A, E and I in 1992, and finally facilities G and H in 1993. Overall, respirator use was recorded for 37% of TPM samples collected in these plants. figure 1 depicts that respirators were used more frequently in some facilities (such as facilities C & E) than others (facilities G & H), which were closely related to facility type (table 2). Indeed, recorded respirator use among TPM measurements was significantly higher in smelting facilities (53%) than fabricating facilities (17%) and the (single) refinery (28%) (P<0.01) (table 2). figure 1 also elucidates that respirators were used not only for samples with high TPM concentrations, but also for those with low TPM concentrations, indicating respirators were probably used to control for exposures other than TPM per se (see Discussion).
Table 2
Respirator use at 12 facilities by number of total particulate matter (TPM) measurements.
Figure 1
Total particulate matter (TPM) measurements and respirator use in TPM samples over time at 12 facilities included in our epidemiologic study. Each data point is a TPM measurement. X-axis is year. Y-axis is natural logarithm of TPM air concentration (in mg/m3). A blue dot indicates that a respirator was not observed to be worn by the worker who wore the TPM sampler (no recorded respirator use); a red dot indicates that a respirator was observed to be worn by the worker who wore the TPM sampler (respirator use was recorded).
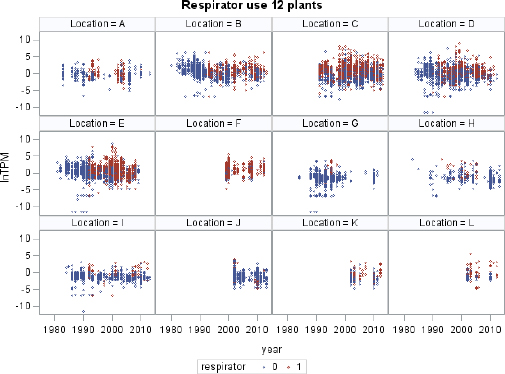
figure 2 presents respirator-use percent by year at all 12 plants [figure 2(a)] and by facility type [figure 2 (b) – (d)]. The respirator use at all facilities (figure 2(a)] revealed an initial increase from no use in 1990 to around 50% of respirator use in 1995, followed by a small increase in the early 2000s. This trend seemed to be mainly driven by the respirator use in smelting facilities [figure 2(b)], which had more substantial increases in 1990s, from no use up to 70%. The increased respirator use in smelting facilities during late 1990s and early 2000s was probably due to the company’s decision to lower the internal occupational exposure limits (OEL), first for polycyclic aromatic hydrocarbons (PAH) in the late 1990s and then for fluorides in the early 2000s. Respirator use in fabricating facilities was not as frequent as it was in smelting facilities [figure 2 (b) and (c) and table 2], with larger variations and a small overall increase over time. Facility A was the only refinery among the 12 facilities. Respirator use at this facility had a lot of variations, probably due to a much smaller number of samples collected, from limited departments and fewer jobs sampled in any given year.
Figure 2
Respirator use by year at (a) all 12 facilities; (b) smelters, (c) fabricating facilities, and (d) the refinery. Each data point is the percent of respirator use that year, defined as the percent of all total particulate matter (TPM) samples collected in that year with respirator use observed during the sample collection. The sample size “n” for each figure is the total number of TPM samples over time in the type of facilities.
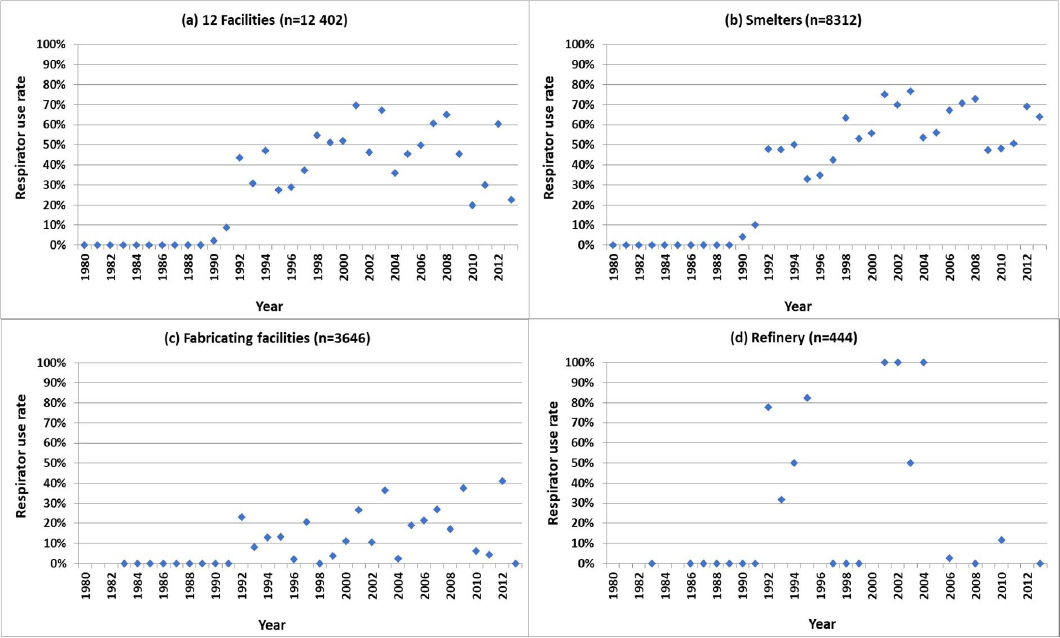
Respirator use at job level
Epidemiologic studies of chronic health outcomes are based on estimates of past exposure for each worker derived by combining employment records of job change with job-based exposure estimates. In order to adjust exposure for respirator use in epidemiologic analysis, we therefore further evaluated respirator use by job. Respirators were used for 170 of 273 jobs in smelting facilities (62%) (table 3). Job titles commonly sampled (N>100 samples) with respirators frequently used (for over 80% of measurements) included “potline operator,” “process control operator,” “bag changer,” “head pot operator,” and “fume-control system attendant” (supplementary table S1). In contrast, respirators were used only for 20% of jobs in fabricating facilities (table 3). Job titles with respirators frequently used in fabrication facilities included “alloy refractory worker,” “torch trimmer,” “alloy maintenance mechanic,” “die welder” and “alloy vacuum furnace operator” (supplementary table S1). Respirators were used for 16 out of 23 jobs at the refinery (70%).
Table 3
Number of jobs affected by respirator use in 12 facilities
Smelters | Fabrication | Refinery | ||||
---|---|---|---|---|---|---|
|
|
|
||||
N | % | N | % | N | % | |
Total number of jobs | 273 | 240 | 23 | |||
Number of jobs for which | ||||||
Respirator ever used | 170 | 62 | 48 | 20 | 16 | 70 |
Respirator never used | 103 | 38 | 192 | 80 | 7 | 30 |
Table 4
Changes in exposure quartile due to respirator use adjustment. “Went down exposure quartile” means changing from higher to lower exposed quartiles; “Went up exposure quartile” means changing from lower to higher exposed quartiles.
Respirator type and style
Mechanical filter respirators accounted for 53% of respirator use among TPM measurements. Chemical cartridge respirators contributed to 26% of respirator use, indicating control for specific chemicals other than particles. Other types included supplied air, powered air, self-contained breathing apparatus and undocumented types. Recorded respirator style included full-face (9%), half-face (79%), quarter-face and helmet/hood.
Impacts of respirator use on TPM exposure
TPM measurements at the 12 facilities (N=12 402) ranged from less than detectable (<0.001 mg/m3) to 8220 mg/m3, with arithmetic mean of 10.6 mg/m3, median of 0.87 mg/m3 and standard deviation of 130 mg/m3. After applying the corresponding APF and WPF to measurements with respirator use, the global arithmetic mean of all TPM measurements reduced to 3.68 and 4.14 mg/m3 for APF- and WPF-adjusted TPM, respectively. When examined by facility type, the overall mean TPM concentration was reduced far more significantly in smelting facilities than fabricating units or the refinery (figure 3a). The average job means decreased from 9.19 mg/m3 to 4.37 (APF-adjusted) and 4.72 mg/m3 (WPF-adjusted) in smelting facilities, whereas it decreased much less in fabricating units and the refinery (figure 3b). Adjusting by APF resulted in significantly lower TPM estimates than adjusting by WPF (P=0.006 in fabrication facilities and P<0.0001 in smelting and refinery facilities). To be conservative in evaluating the effect of respirator use, we used WPF-adjusted estimates henceforth to calculate reductions in estimated TPM exposures.
Figure 3
Total particulate matter (TPM) concentration (mg/m3) before and after respirator use adjustment by facility type: (a) by individual TPM measurements (n=number of TPM measurements); and (b) by jobs (n=number of jobs).
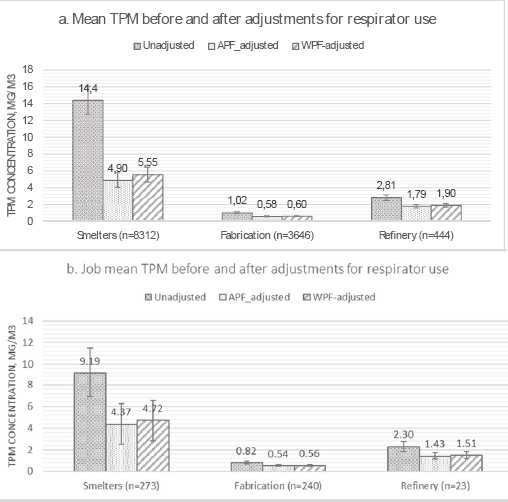
We further evaluated impact of respirator-use adjustment on job exposure “ranking” by examining how many jobs changed exposure quartiles, after adjustment. Among 273 smelting jobs, 89 changed exposure quartile; 48 jobs going up one quartile, 34 going down one quartile and 7 going down two quartiles (table 3). Among 240 fabricating jobs, 20 jobs went up one exposure quartile and 12 went down one or two exposure quartiles.
Discussion
This is the first manuscript to report a review of a large comprehensive industrial hygiene database for respirator use in the aluminum manufacturing industry. Some high TPM concentrations were recorded in the database. Although only accounting for a very small portion of the total number of measurements, these high concentration observations were not rare. For example, among N=12 402 TPM measurements, the maximum was 8220 mg/m3, with 141 measurements >100 mg/m3. The overall respirator use was recorded for 37% of the full shift personal samples collected in these 12 facilities from 1966–2013. Among 141 TPM measurements >100 mg/m3, respirators were recorded to be used for 98 of them (69.5%). It is important to note that TPM was rarely sampled just for itself in industrial hygiene practice; there were typically additional measurements made on a filter sample, suggesting that the sampling targets were other specific airborne chemicals. As a result, respirator-use patterns appeared in TPM data might have been driven by exposure and control of airborne contaminants such as metals, PAH or fluorides. For example, as a fabricating plant, facility L had a very high respirator-use rate. This facility was acquired by the company more recently and had the least number of TPM measurements. Data indicated that all TPM samples at facility L were collected from alloy manufacturing processes and respirators were used there evidently to control exposures to nickel, chromium, cobalt and occasionally silica, styrene and respirable dust. This conjecture is consistent with the observation that respirators were used not only among high concentration TPM samples but also among low concentration ones.
We observed increased respirator use in TPM measurements in the late 1990s and early 2000s, probably due to the company’s lowered internal OEL, first for PAH in the late 1990s and then for fluorides in the early 2000s. An examination on coal tar pitch volatiles (CTPV), benzo(a)pyrene (BaP) and fluorides data revealed increased respirator use among these samples during the aforementioned time periods. For example, respirator use increased from approximately 25% among CTPV and BaP samples before 1995 to >50% after 1995. Similarly, respirator use among fluoride samples increased from <40% to nearly 80% during the late 1990s to early 2000s. Examining respirator use in certain departments, such as “Baked Anode”, “Green Anode” and “Pot Room”, revealed similar patterns, ie, respirator use in these departments increased sharply and shortly after the company lowered internal OEL for the above-mentioned chemicals. Not all observed trends were monotonic possibly due to the implementation of internal standards among facilities over a time period of several years.
Lowering the company’s internal OEL and consequent increase in respirator use led to a substantial reduction in the estimated TPM exposure for some jobs. For example, “bag changer” in smelter had a mean TPM exposure of 73.3 mg/m3 in 1980s when there was no respirator use per database records. In the 1990s, however, although the unadjusted TPM exposure was still high (62.8 mg/m3), the respirator-use adjusted mean TPM concentration reduced to 7.3 mg/m3 (WPF-adjusted). Similarly, TPM exposure for “anode helper” was reduced from 252.1 to 74.6 mg/m3 in 1990 by respirator-use adjustment and further down from 107.4 to 4.6 mg/m3 in the 2000s. Therefore, time trend of respirator use as a consequence of the company lowering its internal OEL appears to have played an important role in TPM exposure; this was likely true for exposures to specific chemical agents as well. Since in this case the respirator use might not be preferentially protecting from TPM exposure, the TPM protection effects of respirators controlling for other airborne contaminants need to be further studied. There were 59 683 TPM measurements in the company’s electronic database, which were collected at 280 facilities between 1966–2013. Respirator use was noted for 36% of these measurements (starting in 1980s), suggesting that the respirator use and potential impact on exposures in other facilities should also be investigated in further studies.
Many studies reported measured respirator performance in the field or simulated WPF (12–18). There were also studies using a distribution of WPF as a parameter in modeling of health risks or respirator performance (19–21), whereas others used respirators as an intervention to evaluate their effects in protection workers’ health in specific industry (22–26). However, respirator use is often not incorporated in exposure estimates in epidemiologic studies evaluating exposure dose–response relationship, due to insufficient information on respirator use in specific industry or among specific study population (2, 27–29). Among the limited studies that did adjust for respirator use, measured WPF was used if they were available, otherwise APF were used (30–36). These protection factors were applied to individual tasks and workers mainly based on questionnaire information (30, 31, 35, 36). Our study appeared to be the first one using a comprehensive industrial hygiene database to estimate TPM exposure that incorporates respirator use. We applied protection factors to individual measurements where respirator use was recorded in the sampling database. The underlying assumption is that the respirator-use percentages among samples recorded in the database were appropriate approximates of workplace compliance rates in a specific facility for a specific job. This overcomes the disadvantage of applying a protection factor uniformly to all measurements of a given job where respirator use was required according to the respirator protection program at a given facility (assuming 100% compliance rate). But this does not rule out the possibility of inappropriate wearing of respirators or poorly fitted respirators, even when respirators were observed to be used. Another potential drawback is applying protection factors for full-shift measurements whereas respirators might be only used during certain tasks. Overestimating the respirator protection may result in underestimation of TPM exposure, which could bias the exposure–response towards higher risk. This could be potentially addressed by performing task-based respirator-use correction. However, adjusting respirator use based on tasks was beyond the scope of the current analysis and requires information on job-task relationship that is not provided in the database. Refined respirator adjustment that incorporates respirator use at task level could be performed in the future study if necessary information is obtained. Moreover, the efficiencies of respirators improved during the decades and correspondingly OSHA APF were adjusted in 1992 and have remained valid for the respirators used currently (30, 37). This historical change in respirator efficiency has little impact on our analysis since respirator use in the facilities examined in the current study started after 1990.
Nevertheless, respirator-use adjustment substantively reduced estimated TPM exposures. An epidemiologic study using unadjusted TPM estimates could potentially overestimate exposure and thus bias the dose-related risk towards the null. This might impact exposure–response relationship more profoundly in smelting facilities than in fabrication facilities. We found respirators were more widely used in smelting than fabrication facilities (52% versus 17% of TPM measurements). Sixty-two percent (62%) of 273 smelting jobs were affected by respirator-use adjustment, while only 20% of 240 were affected in fabricating facilities. Of these, 89 (33%) smelting jobs changed exposure quartiles, either from lower to higher quartile one or vice versa, and some even went down two exposure quartiles (from high to low), whereas, there were only 32 (13%) fabricating jobs that changed exposure quartiles. Since respirators were not only used for high TPM exposure but also for some low TPM exposures – probably due to controlling for other airborne contaminants – respirator-use adjustment had not only reduced exposure at high levels but also changed some low TPM exposures. Our previous epidemiological analysis reported the hazard ratio for CVD was around 1.5 in both smelting and fabricating facilities, though exposure was almost an order of magnitude higher in smelters. It was speculated that the differential exposure–response in smelting and fabrication facilities might be due to differences in TPM composition or healthy worker survivor effect. Subsequent reports confirmed the presence of healthy worker survivor effect operating in the smelters (8). However, based on our adjustment for personal protective equipment, differential respirator use might be an additional explanation as well.