Previous studies of North American industrial sand workers have found elevated mortality from silicosis that was significantly related to cumulative exposure as well as exposure duration and intensity (1–3). The cohort for those studies included 2670 workers at nine sand-producing plants who were employed during 1940–1979. Nested case–control studies to examine exposure-response were based on 37 silicosis and silicotuberculosis deaths, all of which occurred among men who began employment before 1970.
Silicosis mortality declined steadily in the US during the second half of the 20th century, with about 30 deaths occurring annually between 1995–2004 (4). However, evidence of silicosis continues to be found on the chest radiographs of some exposed workers and previous studies have indicated that many cases progress in severity (5–9). Most of these studies examined the prevalence of progression among workers with high exposure concentrations and they provide limited information about how quickly progression occurs or its relationship with exposure.
The current study examined radiographic evidence for silicosis among industrial sand workers in the United States and evaluated its progression among men who continued their employment in the industry. It utilized radiographs and exposure data provided by two companies that have been obtaining serial chest radiographs from employees as part of their health surveillance programs since the 1930s and late 1970s, respectively. Using a nested case–control approach, detailed exposure–response analyses were performed to quantify the relative risk of developing silicosis in relation to the amount of silica exposure. The effect of exposure on the rate of progression was estimated using serial radiographs from workers with silicosis.
Methods
Study population
The study cohort included 1902 male production workers employed between 1938–2003 by two US industrial sand companies at 40 different plants in 22 states. To be included, a worker had to be employed for ≥10 years and have ≥1 posterior-anterior analog chest radiograph obtained ≥10 years after hire as part of the company’s surveillance program. The study was approved by Solutions IRB, a private, fully-accredited Institutional Review Board (approval number 1304030) and the Human Research Protections Office/Institutional Review Board of Tulane University (approval number 13-456812) with waivers of informed consent.
Identification of cases and controls
Potential cases were identified by reviewing each worker’s most recent surveillance radiograph. Two National Institute for Occupational Safety and Health (NIOSH) certified B-Readers independently reviewed and classified the radiographs using the Guidelines for the Use of the ILO International Classification of Radiographs for Pneumoconioses (10). To increase sensitivity in identifying radiographic silicosis, a worker was considered a potential case if both readers classified small opacities at a profusion of ≥1/0, regardless of predominant small opacity shape. If their classifications did not agree, a third NIOSH certified B-reader independently read the radiograph, and the median ILO profusion classification of the three classifications was used to determine if the worker was a potential case.
One of the B-readers then reviewed all available radiographs from each potential case to identify the earliest one with a small opacity profusion ≥1/0. For each potential case, up to three controls were randomly selected from among all men employed at the same plant who were born within three years of the case, and had not been identified as having a small opacity profusion ≥1/0 prior to the date of the case’s earliest radiograph meeting this criterion.
For final determination of radiographic silicosis cases, three experienced NIOSH certified B-readers independently read all radiographs from potential cases that were obtained at least two years apart together with the radiographs from their matched controls having the closest date on or after the date of the case’s first radiograph, with a small opacity profusion ≥1/0. All three B-readers were academic radiologists specializing in thoracic radiology and members of the American College of Radiology Pneumoconiosis Task Force. A total of 1378 radiographs were read in a randomly assigned order and the B-readers were blinded to the worker’s identity, case–control status, exposure and the results from any prior readings. Each radiograph was assessed according to the ILO guidelines (10) and was considered positive for radiographic silicosis if the radiologist classified small opacities with a profusion of ≥1/0 and a predominantly rounded shape occurring in any of the six lung zones and/or identified a large opacity of size A, B or C. The three readings were then compared and the radiograph was defined as positive if ≥2 of the 3 readings met these criteria for radiographic silicosis. Of the 101 potential cases based on small opacity profusion, 68 had ≥1 radiograph meeting the more stringent final criteria for radiographic silicosis.
Exposure assessment
Job histories for cases and controls were provided by the participating companies and included the job title, plant, department or work area, and start and stop dates for each job held while employed by the company, as well as leaves of absence other than vacations. Utilizing >49 000 personal samples of respirable quartz collected by the participating companies, a separate job exposure matrix (JEM) was developed for each plant, beginning with earliest date of hire of participating workers, to the study cutoff date (31 December 2013). Complete details of the methods used to estimate exposure to respirable quartz are described in a separate paper (11). The JEM was linked to the job histories of the participating workers to calculate yearly average exposures concentrations for each individual. Unadjusted exposure estimates, as well as four estimates based on differing assumptions about respirator use and the protection it afforded, were computed.
Statistical analyses
For each set of matched cases and controls, exposure was accumulated from the date of first employment to either the date of radiographic silicosis detection for the case (unlagged exposure) or up to 5, 10 and 15 years prior to that date (lagged exposure). The overall average exposure concentration was computed as the time-weighted average of the exposure concentrations for all jobs held by the worker and net exposure duration was obtained by summing all times during which the worker was exposed. Cumulative exposure, the time-weighted summation of exposure concentrations, was obtained by multiplying the average concentration by net duration.
Conditional logistic regression was used to assess exposure-response relationships between exposure to respirable quartz and the relative risk (RR) of radiographic silicosis. With a nested case–control study design, the odds ratios (OR) from this analysis are unbiased estimates of RR regardless of disease rarity. Exposure–response relationships for cumulative exposure, average concentration and net duration of exposure were assessed in univariate analyses with either the exposure metric or its natural logarithm as a continuous variable. Cumulative exposure was also analyzed as a categorical variable, with cut points based on the quintiles of its combined distribution for the cases and controls. Multivariate analyses with concentration, duration and their interaction (cumulative exposure) were performed to examine whether their contributions to risk were equal and independent. Durations worked at specific ranges of exposure concentration were analyzed together in a multivariate model to assess how the time spent at each exposure level contributed to risk after controlling for the time at all other levels. The possible existence of an exposure threshold was examined by including only those exposures over a specified concentration when computing an exposure metric. The fit of each exposure-response model was evaluated by the likelihood ratio (LR) statistic and the significance of the difference in fit between nested models was assessed by Chi-square test.
Disease progression in terms of changes in small opacity profusion was examined using all readings from sequential chest radiographs obtained on and after the date silicosis was first detected. A numeric code corresponding to the 12-category ILO profusion scale was modeled as a function of time since first detection of radiographic silicosis using random coefficients regression. Potential prognostic factors and their interactions with time were added to the model to assess their effects on the rate of progression. These included (i) exposure, (ii) age, (iii) small opacity profusion at the time of first detection as non-varying covariates, (iv) profusion score on the prior radiograph and (v) average exposure concentration after first detection as time-varying covariates. All models included B-reader as a fixed effect to control for systematic differences in their profusion scores and subject as a random effect to allow for subject-specific differences in the rate of progression. The development of large opacities and its association with exposure were assessed using proportional hazards regression, with time from silicosis detection to the first identification of large opacities by at least two B-readers as the dependent variable.
Results
Case-control analysis
Year of birth for the cases ranged from 1907–1957 (median=1928) and date of hire ranged from 1934–1986 (median=1958). The age at the time radiographic silicosis was first detected ranged from 27.2–64.3 years (median=48.8). One case did not have any eligible controls, leaving 67 cases and 167 matched controls in the case–control analysis. All analyses were performed using both unadjusted exposure estimates, as well as those adjusted for respirator use, but the unadjusted estimates had the strongest associations with risk of radiographic silicosis and only those results are presented. Furthermore, there were relatively slight differences in cumulative exposures, with and without adjustment for respirator use. Cumulative exposures were heavily dependent upon the exposure time prior to about 1970 when the prevailing exposures were much higher than in later years and the workers did not have the benefit of reliable respiratory protection (11).
At the time of the first radiograph having findings indicative of silicosis, exposures were generally higher in the cases than controls but there was considerable variation (table 1) Cumulative exposures for cases ranged from 0.22–25.12 mg/m3 years and average exposure concentrations ranged from 0.015–1.217 mg/m3. For controls, the cumulative exposures ranged from 0.00–16.45 mg/m3 years and average exposure concentrations ranged from 0.000–0.966 mg/m3.
Table 1
Univariate associations between exposure and the relative risk of radiologic silicosis. [OR=odds ratio; CI=confidence interval; SD=standard deviation LR= likelihood ratio.]
Cases | Controls | Coefficient a | OR | 95% CI | P-value | LR statistic | |
---|---|---|---|---|---|---|---|
|
|
||||||
Mean (SD) | Mean (SD) | ||||||
Exposure metric b | |||||||
Cumulative exposure: mg/m3 years | 4.85 (4.18) | 2.41 (2.63) | 0.357 | 1.43 | 1.23–1.66 | <0.0001 | 36.30 |
Average concentration: mg/m3 | 0.28 (0.27) | 0.17 (0.20) | 0.260 c | 1.30 c | 1.11–1.51 | 0.0003 | 13.90 |
Net exposure duration: years | 20.1 (8.9) | 15.9 (10.9) | 0.100 | 1.10 | 1.05–1.16 | <0.0001 | 18.76 |
ln(Cumulative exposure) | 1.15 (1.07) | -0.19 (2.34) | 1.063 | 2.90 | 1.84–4.51 | <0.0001 | 42.43 |
ln(Average concentration) | -1.72 (1.02) | -2.54 (1.65) | 0.749 | 2.11 | 1.42–3.13 | <0.0001 | 23.43 |
ln(Net exposure duration) | 2.95 (0.49) | 2.46 (1.04) | 1.706 | 5.51 | 2.25–13.51 | <0.0001 | 28.21 |
|
|||||||
N (%) | N (%) | Coefficient a | OR | 95% CI | P-value | LR statistic | |
|
|||||||
Cumulative exposure-categorized | |||||||
≤0.50 mg/m3 years | 7 (10.4) | 38 (22.8) | Reference | 1.00 | 41.57 | ||
0.51–1.50 mg/m3 years | 7 (10.4) | 42 (25.1) | 0.092 | 1.10 | 0.26–4.64 | 0.90 | |
1.51–3.00 mg/m3 years | 10 (14.9) | 40 (24.0) | 0.724 | 2.06 | 0.55–7.79 | 0.29 | |
3.01–5.50 mg/m3 years | 20 (29.9) | 26 (15.6) | 2.532 | 12.57 | 2.08–55.47 | 0.0008 | |
>5.50 m3 years | 23 (34.3) | 21 (12.6) | 3.238 | 25.50 | 5.52–117.82 | <0.0001 | |
Average concentration-categorized | |||||||
≤0.05 mg/m3 | 7(10.4) | 45 (27.0) | Reference | 1.00 | 21.45 | ||
0.051–0.100 mg/m3 | 13 (19.4) | 33 (19.8) | 1.388 | 4.01 | 1.14–14.14 | 0.031 | |
0.101–0.200 mg/m3 | 15 (22.4) | 45 (26.9) | 1.337 | 3.81 | 1.13–12.87 | 0.031 | |
0.201–0.400 mg/m3 | 16 (23.9) | 25 (15.0) | 2.259 | 9.58 | 2.52–36.43 | 0.0009 | |
>0.400 mg/m3 | 16 (23.9) | 19 (11.4) | 2.746 | 15.58 | 3.69–65.82 | 0.0002 |
In univariate conditional logistic regression analyses, cumulative exposure, average exposure concentration and exposure duration were all highly associated with risk of radiographic silicosis. The OR indicate a 43% increase in the RR for each 1.0 mg/m3 years increase in cumulative exposure, a 30% increase for each 0.10 mg/m3 increase in average concentration, and a 10% increase for each year of exposure (table 1). The model based on cumulative exposure fit the data better than those based on either average concentration or duration. Both logarithmic transformation and categorization improved the fit of each exposure metric, but cumulative exposure still provided the best fit. When cumulative exposure was analyzed as a categorical variable, the OR for exposures >3.0 mg/m3 years were larger than estimated from the regression with cumulative exposure as a continuous variable. Logarithmic transformation of cumulative exposure yielded RR estimates that more closely approximate the categorical results than those based on the untransformed data (figure 1).
Figure 1
Relative risk estimates from univariate exposure-response models with cumulative exposure as a categorical variable (——), continuous variable (— —) and continuous variable with a natural logarithm transformation (- - - -).
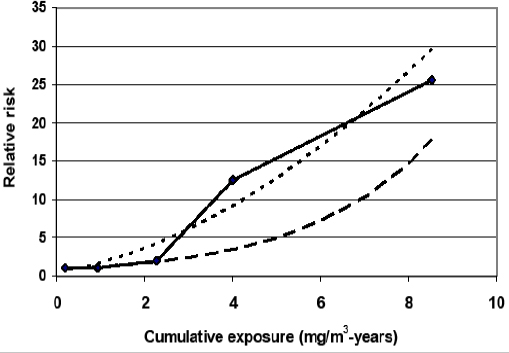
Multivariate analyses with exposure duration and average concentration as separate variables indicated that they do not contribute equally to risk (table 2). When an interaction term was included in the models it was statistically significant, which suggests that the effect of average exposure concentration varies depending on the value of net duration and vice versa. For a cumulative exposure of 2.0 mg/m3 years, RR estimates based on the multivariate models were 2–3 times higher for 20 years of exposure at 0.1 mg/m3 than for 10 years of exposure at 0.20 mg/m3.
Table 2
Multivariate models for the combined effects of exposure concentration and duration on the relative risk (RR) of radiologic silicosis. [SE=standard error; LR=likelihood ratio.]
Coefficient | SE | P-value | LR statistic | Estimated RRa | |
---|---|---|---|---|---|
Average concentration (mg/m3) | 2.959 | 0.823 | 0.0003 | 34.16 | 2.49 |
Net exposure duration (years) | 0.121 | 0.032 | 0.0002 | ||
Average concentration (mg/m3) | -0.361 | 1.552 | 0.816 | 42.5 b | 2.05 |
Net exposure duration (years) | 0.068 | 0.036 | 0.057 | ||
Interaction c | 0.315 | 0.125 | 0.012 | ||
Average concentration (mg/m3) | 3.753 | 1.023 | 0.0002 | 46.49 | 3.23 |
ln(Net exposure duration) | 2.394 | 0.597 | <0.0001 | ||
Average concentration (mg/m3) | -6.629 | 5.269 | 0.21 | 52.30 d | 2.14 |
ln(Net exposure duration) | 1.293 | 0.639 | 0.043 | ||
Interaction | 4.228 | 6.095 | 0.043 |
Exposure concentrations for many of the workers varied substantially over time, so average concentration does not necessarily reflect the actual concentrations they experienced. To examine the effects of exposure at specific concentrations, the length of time spent working at each of six ranges of concentration was computed and included together in a multivariate model (table 3). The OR indicated that after adjustment for time spent working at other concentrations, each year worked at exposure concentrations ≤0.05 mg/m3 increased the RR for radiographic silicosis by 3–5% compared to a year with no exposure, but these increases were not statistically significant. RR increased about 12% per year for exposures between 0.51–4.00 mg/m3 and 43% for each year of exposure at >4.00 mg/m3.
Table 3
Multivariate model for the effects exposures at differing.concentrations on the relative risk of radiologic silicosis. [OR=odds ratio; CI=confidence interval; LR=likelihood ratio]
When exposure–response analyses were repeated using exposure measures that excluded all exposures ≤0.05 mg/m3, the results for cumulative exposure were nearly identical to those shown in table 1. The OR for the association between net exposure duration and risk was also unchanged but the fit to the data was improved. Exclusion of exposures ≤0.05 mg/m3 decreased the OR for average concentration, but the fit to the data was much poorer than when all exposures were used to compute the average.
Analysis of progression
Of the 68 identified cases of radiographic silicosis, 5 had no radiograph after the one detecting radiographic silicosis and were thus excluded from the progression analysis. Of the excluded cases, 1 had a small opacity profusion of 1/0 (median of the three B-Readers), 3 had a profusion of 1/1 and 1 had a profusion of 2/2. The profusion categories at the time of detection for the remaining 63 cases were 1/0 for 52 cases, 1/1 for 4 cases, 1/2 for 2 cases, 2/1 for 2 cases, 2/2 for 2 cases and 3/2 for 1 case. Mean follow-up after the initial radiograph with silicosis was 12 years (range 1.9–28.7) and after excluding radiographs taken <2 years apart, the mean number of subsequent radiographs per case was 5.3 (range 1–12).
Overall, profusion scores after detection of radiographic silicosis increased yearly by 0.078 subcategory, which corresponds to an estimated increase of 1 subcategory every 12.8 years. The rate of increase was inversely related to the profusion score on the radiograph that first showed silicosis (P<0.001). However, this relationship was attributable to one case with a median profusion of 3/2 at detection, who had lower profusion scores on two subsequent radiographs. The estimated progression rates for the other profusion subcategories did not increase or decrease consistently with increasing initial profusion. Similarly, analyses with profusion category on the previous radiograph as a time-varying covariate did not provide evidence that the rate of progression differed across profusion categories.
Age, exposure duration, average concentration and cumulative exposure at the time of first detection of radiographic silicosis were not significantly related to the rate of progression (table 4). However, each 0.10 mg/m3 increase in the average exposure concentration after silicosis detection increased the yearly progression rate by 0.052 over the estimated yearly rate of 0.020 for cases with no subsequent exposure (P<0.001). Hence, the estimated yearly progression for a worker exposed at an average concentration of 0.10 mg/m3 after detection of silicosis is 0.072 subcategory per year. Figure 2 shows the estimated progression from an initial profusion of 1/0 for workers with differing average exposure concentrations following the date of first detection.
Table 4
Effects of age and exposure on the rate of progression after silicosis detection. [SE=standard error.]
Coefficient a | SE | P-value | |
---|---|---|---|
Age at detection: years | -0.001 | 0.004 | 0.727 |
Cumulative exposure up to detection: 1 mg/m3 years | -0.003 | 0.006 | 0.668 |
Average concentration up to detection: 0.10 mg/m3 | -0.003 | 0.009 | 0.777 |
Exposure duration up to detection: 10 years | 0.005 | 0.037 | 0.900 |
Average concentration since detection: 0.10 mg/m3 | 0.052 | 0.014 | <0.001 |
Figure 2
Estimated progression of small opacity profusion for workers exposed at concentrations of 0.025 mg/m3 (——), 0.05 mg/m3 (— —), 0.10 mg/m3 (- — -), and 0.20 mg/m3 (- - - -) after detection of silicosis.
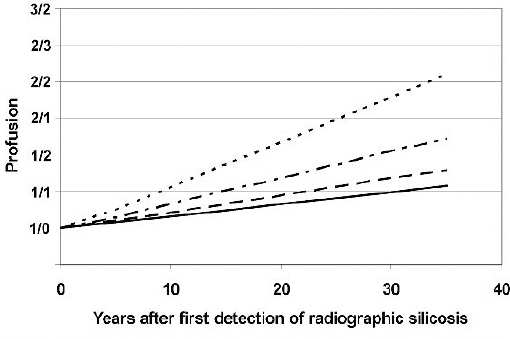
Twenty-nine workers had large opacities identified on their last radiograph by at least two of the three B-Readers (17 size A, 10 size B and 2 size C based on the median of three readings). For three of these workers, size A large opacities were present on the first radiograph meeting the criteria for silicosis. For the remaining workers, large opacities appeared 2.0–20.8 years after silicosis detection (mean 7.1 years). Time to the first appearance of large opacities was not related to exposure either before or after silicosis detection but workers who had an increase in small opacity profusion during follow-up were more likely to develop large opacities (hazard ratio 2.09, 95% CI 1.01–4.49).
Discussion
This study found that the occurrence of radiographic silicosis in US industrial sand workers was quantitatively related to cumulative exposure, exposure duration, and average exposure concentration. Multivariate exposure-response models that allowed differing effects for net exposure duration and average concentration indicated that if two workers of the same age have the same cumulative exposure, the one with longer exposure will be at higher risk of developing silicosis, even though his average exposure concentration is lower. However, all cases and their controls were employed at the time silicosis was first detected in the case and there were few gaps in exposure, so net exposure duration was virtually the same as time since first exposure for many of the workers. It was therefore impossible to determine if the workers with shorter exposure at higher average concentrations had lower risk because of their shorter time since first exposure.
Univariate analyses indicated that workers with average exposures concentrations between 0.051–0.10 mg/m3 had a substantially higher risk of silicosis than workers with lower average concentrations. However, workers in the current study were exposed at varying concentrations during the course of their working tenures and none of the exposure metrics reflect this variation. Differences in exposure patterns over time are likely to influence risk, particularly for workers with low average concentrations who were exposed at higher levels early in their employment. Thus, the silicosis risk associated with a specific average concentration may not be applicable to workers consistently exposed at that level. Multivariate analysis of exposure durations at various concentrations indicated that the time spent working at 0.051–0.10 mg/m3, significantly contributed to risk after adjustment for exposures at higher concentrations, while the time worked at exposure ≤0.05 mg/m3 did not. However, the difference in risk was not nearly as large as suggested by the results based on average concentration. It is unclear if exposures ≤0.05 mg/m3 or ≤0.10 mg/m3 would be associated with radiographic silicosis among individuals who were never exposed at higher concentrations. Determination of this would require long-term follow-up of workers exposed at consistently low concentrations.
Very few exposure–response studies for silicosis have looked at the individual effects of exposure concentration and duration. In a cross-sectional study of 100 Colorado hard rock miners, Kreiss & Zhen (12) examined a number of exposure–response models, including one with years of silica exposure, average concentration and years since last exposure. Their coefficient for years of exposure (0.114) was similar to that in the current study for the bivariate model with duration and concentration (0.121). However, their coefficient for average concentration was much higher (40.149 versus 2.595 per 1 mg/m3). The two studies differ fundamentally in that Kreiss & Zhen analyzed silicosis prevalence 0–68 years after last exposure and computed the average concentration overall years of employment. In contrast, the current study analyzed incident cases of silicosis occurring during employment and computed the average exposure up to the time silicosis was first detected, which would generally be higher than the average at a later date because of decreases in exposure levels over time. Exposure concentrations in the Kreiss & Zhen study may also have been substantially underestimated because they were based on measurements taken from 1974–1982 and there was no adjustment for secular trend, even though some exposures occurred as early as the 1920s.
Hughes et al (13) used serial radiographs from 2342 workers employed in a diatomaceous earth mining and processing facility to identify incident cases of silicosis. The radiographs were obtained primarily during employment as part of a voluntary surveillance program and the number of available radiographs varied widely among workers. Hughes et al did not analyze risk as a function of exposure duration and average concentration but rather examined the relationship between cumulative exposure and silicosis risk among workers with average concentrations ≤0.50 mg/m3 and >0.50 mg/m3. In contrast to the current study, they found that workers with higher average concentrations were at greater risk than those with the same cumulative exposure but lower concentrations and hence longer exposure. It is unclear why the two studies produced such different results, but only 16% of the industrial sand workers who developed silicosis had average exposures >0.50 mg/m3, compared to 72% of the diatomaceous earth workers. In addition, none of the silicosis cases among industrial sand workers with high average exposure concentrations had cumulative exposures <4.0 mg/m3 years but a substantial number of the diatomaceous earth workers did. Co-exposure to asbestos in diatomaceous earth workers may have contributed to these differences. It is also possible that more workers in the Hughes et al study had been exposed to silica during previous employment because some cases occurred as soon as a month after hire.
Accurate characterization of exposure–response is important for valid estimation of the risk associated with long-term exposure at fixed concentrations, which is the most relevant information for determining permissible exposure limits. The US Occupational Safety and Health Administration estimated the number of silicosis cases per 100 workers with 45 years of silica exposure at a concentration 0.1 mg/m3 for each of nine published studies that included serial radiographs to identify evidence of silicosis and examined its relationship to exposure (14). The estimates ranged from 1.2 for Ontario hard rock miners (15) to 77 for South African gold miners (16). There are a number of potential reasons for these varying results, including differences in the physicochemical properties of the crystalline silica dusts from different industrial settings, which can influence biological response (17). Another factor not usually considered is that all of the studies included workers with widely ranging exposure durations and concentrations, and exposure–response was examined in terms of cumulative exposure. The results from the current study indicate that differences in the distributions of exposure durations and concentrations between cohorts could have contributed substantially to the observed differences in the relationship between cumulative exposure and silicosis risk.
The current study also found that the rate of progression across small opacity profusion categories was associated with a worker’s average exposure concentration after silicosis detection but not the duration, concentration or cumulative exposure experienced up to that time. These results underscore the importance of regular surveillance to detect radiographic findings indicative of silicosis and management of subsequent exposure to minimize progression. The estimated progression rate of 0.072 profusion subcategory per year for a person with an average exposure of 0.10 mg/m3 after silicosis detection indicates that it would take him >20 years to progress from a profusion of 1/0 to 1/2.
Although ILO profusion categories are a semi-quantitative measure of disease progression, they were assigned a numeric score and treated as a quantitative measure in the progression analysis because there was little evidence that the rate of progression was related to profusion category. This approach was also selected based on preliminary analyses indicating that there were systematic differences in the profusion scores from the three B-readers but not in the progression rates based on their scores. There was also considerable fluctuation in serial profusion scores within the same worker because all radiographs were randomly ordered and read blindly, so the B-readers could not compare sequential radiographs. By analyzing profusion score as a quantitative measure, it was possible to control for these sources of variability and obtain a general estimate of the effect of exposure on the speed of progression across profusion categories. It should be noted however that 60% of the silicosis cases in the study had average exposures <0.10 mg/m3 and only 8 (13%) had exposures >0.20 mg/m3 after detection of silicosis. Therefore, the estimated effect of exposure on progression rate may not be applicable at higher concentrations.
Development of large opacities was not significantly associated with exposure received either before or after silicosis detection. Determination of the presence of large opacities was based on agreement between at least two of the three B-readers and there was considerable variability in the assessments both among readers and across serial radiographs. This variability made it difficult to accurately determine when large opacities first appeared, which may have limited the ability to detect associations with exposure. Development of large opacities was associated with an increase in small opacity profusion, providing validation for the use of profusion as a marker of progression.
Other studies of progression have compared radiographs at ≥2 time points to determine the percent of silicosis cases that progressed and only a few have related this to exposure. Dumavibhat et al (18) examined time to first progression using serial radiographs from Japanese tunnel workers who received treatment at a clinic after discontinuing their employment. They reported a median of 17 years for the time to the first increase in profusion, which was not related to exposure duration. The relationship between the exposure concentration and the probability of silicosis progression has been examined in a study of granite quarry workers, most of whom were no longer being exposed to silica (6), and a study of current and recent sandblasters (5). These studies found that the likelihood of any progression was related to both the total duration of exposure and average concentration. Neither of these studies examined the effects of exposures before and after silicosis detection.
All results presented in this report are based on exposure estimates that were unadjusted for respirator. Very similar results were obtained when the exposures were adjusted for respirator use and efficiency, but the regression coefficients were slightly larger. For example, in the exposure–response analysis the regression coefficient for cumulative exposure based on observed respirator use for each job is 1.075 times larger than that for no respirator use if a workplace protection factor (WPF) of 5 is assumed and is 1.086 times larger if a WPF of 10 is assumed. The adjusted exposures provided poorer fit with the data, which is likely due to the additional variability introduced by incorporating respirator use into the exposure estimates. Estimated use was based on year and job but likely varied among individuals with the same job. Cumulative exposure computed by assuming continuous respirator use is perfectly correlated with cumulative exposure based on no respirator use and thus provided an identical fit to the data but the regression coefficients are 5 and 10 times larger for WPF of 5 and 10, respectively.
This study had several limitations. As in most studies of historical cohorts, there were uncertainties in some of the exposure estimates. These potential errors likely affected the cases and controls non-differentially, so they would not be expected to substantially impact the exposure–response results. In addition, some of the workers were employed at plants that had been in operation under different ownership before being acquired by the companies participating in this study. Detailed job histories prior to acquisition were not always available for these workers and they were assigned the average exposure concentrations for the plant for these time periods (11). Cases and controls had similar amounts of missing job data because they were matched on plant and birth year. Although this likely reduced potential bias in the exposure–response results due to the missing information, it added variability to the exposure estimates. Also, as noted above, time since first exposure was confounded with duration of exposure, precluding estimation of their individual effects. Finally, smoking information was not available for the workers, so its effect on the development and progression of silicosis could not be investigated.