Studies suggest that flight attendants have an increased incidence of breast cancer compared to the general population (1, 2). However, the reason is unclear. Flight attendants may be at an increased risk of breast cancer because of workplace exposure to elevated levels of cosmic radiation or circadian disruption from traveling across multiple time zones and working at night (3) or because of difference in non-occupational risk factors for breast cancer, such as parity and age at first birth, which may be associated with work as a flight attendant.
We previously reported that parity and age at first birth may largely explain an observed excess in breast cancer incidence among a large cohort of US female flight attendants formerly employed by Pan American World Airways (Pan Am) (4). Breast cancer incidence was not significantly associated with cosmic radiation dose or measures of circadian rhythm disruption [cumulative time zones crossed and cumulative time spent working during the standard sleep interval (SSI); ie, between 22:00–08:00 hours at the flight attendant’s home base domicile] except among women with parity of ≥3. However, we only considered three potential confounders or effect modifiers (parity, age at first birth, and family history of breast cancer) by stratification, and we did not consider these potential confounders simultaneously, nor did we conduct quantitative exposure–response modeling.
In the current study, we further examined associations of breast cancer incidence in this cohort by considering a wider range of potential confounders and effect modifiers, time windows of exposure, and various model forms. This is the first large cohort study of breast cancer in flight attendants with comprehensive data on non-occupational risk factors for breast cancer.
Methods
Cohort description
The study cohort is a subset of a mortality cohort (5) assembled from company personnel records that included 1707 men and 9617 women who worked for ≥1 year as a flight attendant before Pan Am ceased operation in 1991, were US citizens when hired, and worked ≥1 day after 1 January 1953.
The cohort inclusion criteria and follow-up are described in detail elsewhere (4). Briefly, women in the mortality cohort with a last known address in the US (N=9512) or their next-of-kin, if deceased, were invited to participate in the current study by completing a telephone interview or mailed questionnaire. Of those, 6093 (64.4% of eligible women) completed the interview, gave informed consent, and were free from breast cancer at the start of follow-up and thus were included in the study. Data on participants and non-participants are provided in the Appendix, supplementary table S1 (www.sjweh.fi/index.php?page=data-repository).
Interview and cancer registry data
Data on demographics, work history, risk factors for breast cancer, and cancer diagnoses were obtained in the interview. The interview data were supplemented with data on race, education, height, and weight in the company records and used to create analysis variables for covariates as described in the Appendix. Breast cancer cases, including carcinoma in situ, were identified by interview, medical record follow-back for reported cancers, and linkage with cancer registries in six states (California, Florida, New York, Washington, Texas, and Virginia) where many cohort members had been based or lived. Because of the high positive predictive value of self-reported breast cancer (6), all breast cancer cases identified from the interview were included in the analysis except for two that were refuted by medical records.
Exposure assessment
Individual, time-dependent estimates of cosmic radiation absorbed dose, SSI travel, and number of time zones crossed were developed by linking work history data with domicile- and era-specific exposure estimates as described elsewhere (4, 7). Briefly, domicile- and era- specific absorbed dose rates were derived from flight schedule data and cosmic radiation absorbed dose per flight calculated using the CARI-6P computer program. For most self-respondents, absorbed dose (in mGy without radiation or tissue weighting factors applied) received during work flights while employed as a flight attendant by any airline and commuter flights (flights taken to the domicile to which the flight attendant was assigned) was estimated by linking domicile- and/or era-specific absorbed dose rates with work history data obtained from interviews. SSI travel and number of time zones crossed were estimated in an analogous way using methods described elsewhere (8, 9). For 195 cohort members without self-reported work history data from interviews, absorbed dose, SSI travel, and number of time zones crossed incurred during employment as a flight attendant at Pan Am and National airlines (which was purchased by Pan Am in 1981) only were estimated as described by Waters et al (9) using work history data obtained from the company records.
Statistical analyses
Full risk sets were created and analyzed in Cox proportional hazards models with an age timescale to evaluate breast cancer risk with the exposure metrics (evaluated one-at-a-time due to high observed correlations) using the SAS PHREG procedure (SAS Institute Inc, Cary, NC, USA) and the Epicure PECAN module (Risk Sciences International, Ontario, Canada). Women were followed from the date the mortality cohort criteria were met or, for those who transferred to Pan Am from National, the transfer date, whichever was later, until the date of death, diagnosis of first breast cancer, or the interview, whichever came first. Risk sets included the case and all cohort members who entered the cohort prior to the case’s age at diagnosis and survived without breast cancer to at least that age. Cumulative exposures were lagged by ten years and truncated at the index case’s diagnosis age. As is standard in such analysis, women whose exposures lagged to zero were assigned zero cumulative exposure.
Log-linear, power (log-linear model using a log transformation of the exposure variable), and linear excess relative risk (ERR) (10) model forms were considered. Model fit was evaluated using Akaike Information Criterion (AIC) (11) and restricted cubic spline (12) and categorical (cut-points based on exposure quintiles among cases) models. For power model analyses, one-third of the smallest positive exposure (0.777 μGy, 0.018 hours, and 0.028 time zones crossed) was used for exposures that lagged to zero to avoid taking the natural logarithm of zero. Exposure windows defined by age and reproductive periods were also evaluated in log-linear models. Hazard ratios (HR) and ERR were estimated based on maximum partial likelihood, significance tests were based on the likelihood ratio test (LRT), and confidence intervals (CI) were based on the profile likelihood.
All final models were adjusted for age (the time scale) and included one cumulative exposure metric in addition to the following covariates: menarche age, height, alcohol consumption status, parity, age at first birth, menopausal status, hormone replacement therapy use, and family history of breast cancer among first and/or second degree relatives. These covariates were included in the final models regardless of whether they were associated with breast cancer risk in the cohort because they are well-known risk factors for breast cancer. Effect modification for each of these covariates was evaluated separately in models with the best-fitting form by including the appropriate product term and comparing models with and without the product term using the LRT. All remaining covariates were included in the models as potential confounders. Effect modification was evaluated because parity, age at first birth, and family history of breast cancer have been shown to be potential effect modifiers in studies of radiation-induced breast cancer (13), and other covariates in the final models have been shown to be potential effect modifiers in other studies of breast cancer (eg, 14–16). The proportional hazards assumption was then evaluated for each exposure metric by including an interaction term between age and each exposure metric in the final models and testing for significance using the LRT.
Other covariates that were evaluated but not included in the final models because they were not associated with breast cancer in the cohort (P>0.25) and were not significant predictors of breast cancer risk that improved model fit when added one at a time to models with the covariates listed above are shown in table 1.
Table 1
Covariates evaluated in the analysis
Covariate | Fixed variable | Time- dependent variable | Included in final model |
---|---|---|---|
Menarche age | ✓ | ✓ | |
Height | ✓ | ✓ | |
Alcohol consumption status a | ✓ | ✓ | |
Parity | ✓ | ✓ | |
Age at first birth | ✓ | ✓ | |
Menopausal status | ✓ | ✓ | |
Hormone replacement therapy use a | ✓ | ✓ | |
Family history of breast cancer b | ✓ | ✓ | |
Birth year | ✓ | ||
Race/ethnicity | ✓ | ||
Education | ✓ | ||
Jewish religion c | ✓ | ||
Birthplace d | ✓ | ||
Body mass index at age 20 | ✓ | ||
Change in body mass index e | ✓ | ||
Cigarette smoking status a | ✓ | ||
Pack-years of smoking a | ✓ | ||
Average number of alcoholic drinks per week a | ✓ | ||
Cumulative number of alcoholic drinks a | ✓ | ||
Breastfeeding status | ✓ | ||
Breastfeeding duration | ✓ | ||
Age at menopause | ✓ | ||
Age at natural menopause | ✓ | ||
Personal history of breast biopsy for benign breast disease | ✓ |
a Because events leading to a breast cancer diagnosis may have caused some cohort members to stop using cigarettes or alcohol or to discontinue hormone replacement therapy, these variables were lagged by two years.
c Jewish religion was evaluated because women of Ashkenazi Jewish descent are more likely to have BRCA1 and BRCA2 gene mutations and thus, may have an increased breast cancer risk.
Finally, sensitivity analyses were conducted to: (i) evaluate alternative lag periods (5, 15, and 20 years), (ii) exclude cohort members for whom interview data were provided by proxy respondents (N=134), (iii) exclude cohort members who had ≥1 self- or proxy-reported diagnostic examinations or radiation treatments before a breast cancer diagnosis that would result in >100 mGy absorbed dose to the breast (N=82), and (iv) include a time-dependent term to indicate cohort members who underwent a hysterectomy without a bilateral oophorectomy before menopause or age 50 years, the mean age of natural menopause in the cohort, whichever was earlier (N=405). The fourth sensitivity analyses was conducted because some of these women may have been pre-menopausal but menopausal status was based on whether each subject had a menstrual period within the last 12 months, resulting in possible misclassification for this risk factor. For the power model analysis, sensitivity analyses were also conducted to: (i) use alternative values (the smallest positive exposure or the 0.05 percentile of positive exposures) for exposure estimates that lagged to zero or less than the 0.05 percentile of positive exposures, respectively, and (ii) to add one-third of the smallest positive exposure and these alternative values to all exposures instead of using these values for exposures that lagged to zero or less than the 0.05 percentile of positive exposures. The 0.05 percentile was considered because the minimum positive lagged exposure value was very low compared to other exposure values.
The Institutional Review Boards of the National Institute for Occupational Safety and Health and the National Cancer Institute approved the study.
Results
Descriptive information for the 344 women with breast cancer and 5749 women without breast cancer in the study is shown in table 2. Breast cancer risk in the cohort according to non-occupational factors in the final models is shown in table 3. Breast cancer risk according to other factors that were evaluated but not included in the final models is shown in the Appendix, supplementary table S2 (www.sjweh.fi/index.php?page=data-repository).
Table 2
Characteristics of women with and without breast cancer. [BMI=body mass index; IQR=interquartile range; SSI=standard sleep interval (ie, 22:00–08:00 hours at the flight attendant’s home base domicile).]
Characteristic | Breast cancer cases (N=344) | Non-cases (N=5749) | ||||||||
---|---|---|---|---|---|---|---|---|---|---|
|
|
|||||||||
N | % | Mean | Median | IQR | N | % | Mean | Median | IQR | |
Proxy respondent | 33 | 10 | . | . | . | 101 | 2 | . | . | . |
Year of birth | . | . | 1943 | 1944 | 1939–1947 | 1949 | 1947 | 1944–1954 | ||
White, non-Hispanic | 303 | 88 | . | . | . | 4870 | 85 | . | . | . |
Education | ||||||||||
High school or less | 15 | 4 | . | . | . | 285 | 5 | . | . | . |
Some college or professional training | 112 | 33 | . | . | . | 1701 | 30 | . | . | . |
College graduate | 143 | 42 | . | . | . | 2432 | 42 | . | . | . |
Post-college graduate work | 73 | 21 | . | . | . | 1324 | 23 | . | . | . |
Unknown | 1 | <1 | . | . | . | 7 | <1 | . | . | . |
Age at menarche (years) a | . | . | 12.8 | 13 | 12–13 | . | . | 13.0 | 13 | 12–14 |
Height (cm) a | . | . | 166 | 165 | 163–170 | . | . | 166 | 165 | 163–170 |
BMI at age 20 years (kg/m2) a | . | . | 20 | 20 | 19–21 | . | . | 20 | 20 | 19–21 |
BMI change from 20 years (kg/m2) a | . | . | 3.4 | 3.0 | 0.8–5.3 | . | . | 3.5 | 2.7 | 1.0–5.1 |
Ever smoker a, b | 183 | 53 | . | . | . | 2970 | 52 | . | . | . |
Pack-years among smokers b | . | . | 15 | 10 | 2.3–20 | . | . | 12 | 6.5 | 1.6–18 |
Ever drank alcohol a, b | 295 | 86 | . | . | . | 4741 | 82 | . | . | . |
Among ever drinkers: | ||||||||||
Average drinks/week b | . | . | 5.8 | 4.0 | 2.0–7.0 | . | . | 5.2 | 4.0 | 2.0–7.0 |
Cumulative drinks b | . | . | 7500 | 4500 | 2000–10000 | . | . | 7400 | 4400 | 1800–9600 |
Parity b | ||||||||||
0 | 124 | 36 | . | . | . | 2001 | 35 | . | . | . |
1–2 | 174 | 51 | . | . | . | 2834 | 49 | . | . | . |
≥3 | 42 | 12 | . | . | . | 842 | 15 | . | . | . |
Unknown | 4 | 1 | . | . | . | 72 | 1 | . | . | . |
Number of births among parous women b | . | . | 2.0 | 2.0 | 1–2 | . | . | 2.0 | 2.0 | 1–2 |
Age at first birth (years) b | . | . | 30 | 29 | 26–33 | . | . | 30 | 30 | 27–34 |
Ever breast-fed, among those with ≥1 live birth(s) b | 168 | 78 | . | . | . | 3044 | 83 | . | . | . |
Age first breast-fed (years) b | . | . | 31 | 31 | 28–34 | . | . | 31 | 30 | 28–34 |
Breastfeeding, weeks b | . | . | 50 | 35 | 18–66 | . | . | 62 | 48 | 22–83 |
Menopausal status b | ||||||||||
Pre-menopausal | 135 | 39 | . | . | . | 2037 | 35 | . | . | . |
Menopausal – natural | 106 | 31 | . | . | . | 2539 | 44 | . | . | . |
Menopausal – other | 86 | 25 | . | . | . | 1050 | 18 | . | . | . |
Unknown/indeterminate | 17 | 5 | . | . | . | 123 | 2 | . | . | . |
Age at menopause, among menopausal (years)a, b | . | . | 46 | 48 | 44–50 | . | . | 48 | 50 | 45–52 |
Hormone replacement therapy b | ||||||||||
Never used | 201 | 58 | . | . | . | 2878 | 50 | . | . | . |
Current user | 79 | 23 | . | . | . | 1564 | 27 | . | . | . |
Recent user (quit ≤2 years ago) | 37 | 11 | . | . | . | 689 | 12 | . | . | . |
Distant user (quit >2 years ago) | 15 | 4 | . | . | . | 548 | 10 | . | . | . |
Unknown/indeterminate | 12 | 3 | . | . | . | 70 | 1 | . | . | . |
Years of use, among ever users b | . | . | 7.4 | 6.8 | 3.0–10 | . | . | 7.5 | 6.1 | 3.0–11 |
Family history of breast cancer in first and/or second degree relative a, b | 149 | 43 | . | . | . | 1904 | 33 | . | . | . |
History of benign breast disease a, b | 68 | 20 | . | . | . | 944 | 16 | . | . | . |
Age at first exposure (years) c | . | . | 23 | 22 | 22–24 | . | . | 23 | 22 | 22–24 |
Year of first exposure c | . | . | 1966 | 1967 | 1962–1970 | . | . | 1972 | 1970 | 1966–1978 |
Years since first exposure c | . | . | 36 | 36 | 33–41 | . | . | 31 | 33 | 25–37 |
Duration of exposure (years) b, d | . | . | 11 | 6.8 | 3.1–16 | . | . | 12 | 7.7 | 3.1–17 |
Cumulative cosmic radiation absorbed dose (mGy) b | . | . | 10 | 5.5 | 2.6–16 | . | . | 12 | 7.3 | 2.7–17 |
Cumulative SSI travel (hours) b | . | . | 2000 | 1300 | 520–3000 | . | . | 2200 | 1500 | 510–3200 |
Cumulative time zones crossed (N) b | . | . | 5000 | 2700 | 1200–7400 | . | . | 5900 | 3700 | 1300–8700 |
a Age at menarche missing for 270 subjects (including all subjects with proxy respondents), height missing for 1 subject, body mass index missing for 5 subjects, change in body mass index missing for 56 subjects, smoking status missing for 29 subjects, alcohol consumption status missing for 28 subjects, age at menopause missing for 3 subjects, family history of breast cancer missing for 569 subjects, and history of benign breast disease missing for 70 subjects.
b Cumulative value calculated as of the date of diagnosis for cases and as of the interview date for non-cases.
c First exposure was based on first employment as a flight attendant in jobs involving flying and thus incurring exposure to cosmic radiation and circadian rhythm disruption.
d Duration of employment as a flight attendant in jobs involving flying; all participants were employed for one or more years as a flight attendant according to the personnel records, but 24 participants reported less than one year of employment as a flight attendant in jobs involving flying in the interview.
Table 3
Association between reproductive and other factors included in the final models and breast cancer incidence in the cohort. [HR=hazard ratio; 95%CI=95% confidence interval.]
HR a | 95% CI | |
---|---|---|
Age at menarche | ||
<12 years | 1.44 | 1.01–2.05 |
12–13 years | 1.12 | 0.86–1.48 |
≥14 years | 1 | |
Don’t know | 2.78 | 1.76–4.30 |
Height (1 cm increase b) | 1.02 | 1.00–1.04 |
Alcohol status (lag=2 years) | ||
Non-drinker | 1 | |
Current | 1.18 | 0.87–1.63 |
Former | 1.50 | 0.95–2.35 |
Parity | ||
0, 1, 2 | 1 | |
≥3 | 0.71 | 0.49–1.00 |
Age at first birth (years) | ||
Nulliparous | 1 | |
<30 | 1.23 | 0.93–1.63 |
30–<35 | 1.14 | 0.84–1.54 |
≥35 | 1.30 | 0.90–1.85 |
Menopausal status | ||
Pre-menopausal | 1 | |
Natural menopause | 1.28 | 0.92–1.80 |
Other menopause | 1.76 | 1.25–2.46 |
Don’t know | 1.06 | 0.54–1.95 |
Hormone replacement therapy use (lag=2 years) | ||
Don’t know | 1.35 | 0.62–2.66 |
Never or 2-year lag duration = 0 | 1 | |
2-year lag duration <3 years | 0.70 | 0.45–1.04 |
2-year lag duration 3–<7 years | 1.01 | 0.67–1.47 |
2-year lag duration 7–<10 years | 1.50 | 0.93–2.34 |
2-year lag duration ≥10 years | 0.90 | 0.54–1.44 |
Family history of breast cancer | ||
1st and/or 2nd degree relative | 1.65 | 1.31–2.07 |
Neither | 1 | |
Don’t know | 1.18 | 0.81–1.68 |
The relation of breast cancer incidence with each exposure metric was modified by parity. Results among women with parity of 0, 1, and 2 were similar and non-significant (data not shown), so these categories were collapsed, which improved model fit. Results for the linear ERR model, which overall appeared to be best-fitting, are shown in table 4. Significant positive exposure–response relations among women with parity of three or more were observed for absorbed dose and time zones crossed (ERR 1.6 per 10 mGy, 95% CI 0.14–6.6 and 1.5 per 4600 time zones crossed, 95% CI 0.14–6.2, respectively). Similar exposure–response trends were observed for SSI travel (ERR 0.99 per 2000 hours, 95% CI -0.041–4.3), but were not statistically significant. Similar results were obtained for other model forms [see Appendix, supplementary table S3 (www.sjweh.fi/index.php?page=data-repository) and figure 1] and in all sensitivity analyses. HR among women with parity of ≥3 were similar across birth windows for absorbed dose and time zones crossed but the HR was higher for time spent working during SSI travel at first birth or later than for SSI travel prior to the first birth. The HR among women with parity of ≥3 was higher for all three exposures accrued at age ≥40 years than for exposures accrued at younger ages, but the CI were wide and overlapped. There was no evidence of effect modification by age at menarche, height, use of hormone replacement therapy, or family history of breast cancer (results not shown). Findings for alcohol consumption status and menopausal status were not robust, but depended on the model form (results not shown; see www.sjweh.fi/index.php?page=data-repository for additional information).
Table 4
Excess relative risk (ERR) of breast cancer for 10-year lagged cumulative exposure variables stratified by parity (0, 1, 2 versus ≥3 births).a [AIC=Akaike Information Criterion; 95% CI=profile-likelihood based 95% confidence interval.]
Cumulative exposure metric (comparison) | AIC | Parity (0, 1, 2) | Parity (≥3) | P-valueb | ||
---|---|---|---|---|---|---|
|
|
|||||
ERR | 95% CI | ERR | 95% CI | |||
Absorbed dose | ||||||
10 mGy increase | 5364.405 | -0.021 | -0.14–0.17 | 1.6 | 0.14–6.6 | 0.02 |
Standard sleep interval travel | ||||||
2000 hour increase | 5366.195 | -0.039 | -0.15–0.14 | 0.99 | -0.041–4.3 | 0.06 |
Time zones crossed | ||||||
4600 zone increase | 5364.416 | -0.0017 | -0.12–0.18 | 1.5 | 0.14–6.2 | 0.02 |
a 6001 subjects including 340 cases were included in the analysis; all models excluded subjects with missing height, alcohol status, or birth information. Results are adjusted for age (since risk sets were created based on attained age), age at menarche, height, alcohol status, age at first birth, menopausal status, use of hormone replacement therapy, and family history of breast cancer (covariate categories are indicated in table 3). The models also included terms for parity, exposure, and the product of parity and exposure. Specifically, under the stratified model, the hazard function for the jth individual in the ith risk set is expressed asλij(t) = λi0(t) exp[Z’ijβ]. For covariates X1, …, Xp (assume Xp is an indicator of ≥3 births versus 0/1/2 births) and exposure variable Xe, the linear excess risk model is given byλij(t) =λi0(t) exp[β1X1 + … + βpXp] (1+ βeXe + βpeXpXe). For exposure D, the ERR is βe × D for parity 0/1/2 and the ERR is (βe+βpe) × D for parity ≥3 (where relative risk = 1 + ERR).
Figure 1
Breast cancer hazard ratios from the parity interaction models (curves on the left-hand-side reflect risk among those with <3 births; curves on the right-hand-side reflect risk among those with ≥3 births). Horizontal lines represent the quintile model; the solid curve with dots (●) the log-linear model; the dashed curve with squares (◾) the power model; the solid curve with diamonds (♦) the spline model; and the dashed curve with triangles (▴) the excess relative risk model. Hazard ratios are adjusted for attained age, age at menarche, height, alcohol use, age at first birth, menopausal status, hormone replacement therapy use and family history of breast cancer and are normalized to the mean (0.78 mGy of absorbed dose, 150 hours of standard sleep interval travel, and 360 time zones crossed) of the baseline group in the categorical models. The models also included terms for parity, exposure, and the product of parity and exposure. [SSI=standard sleep interval.]
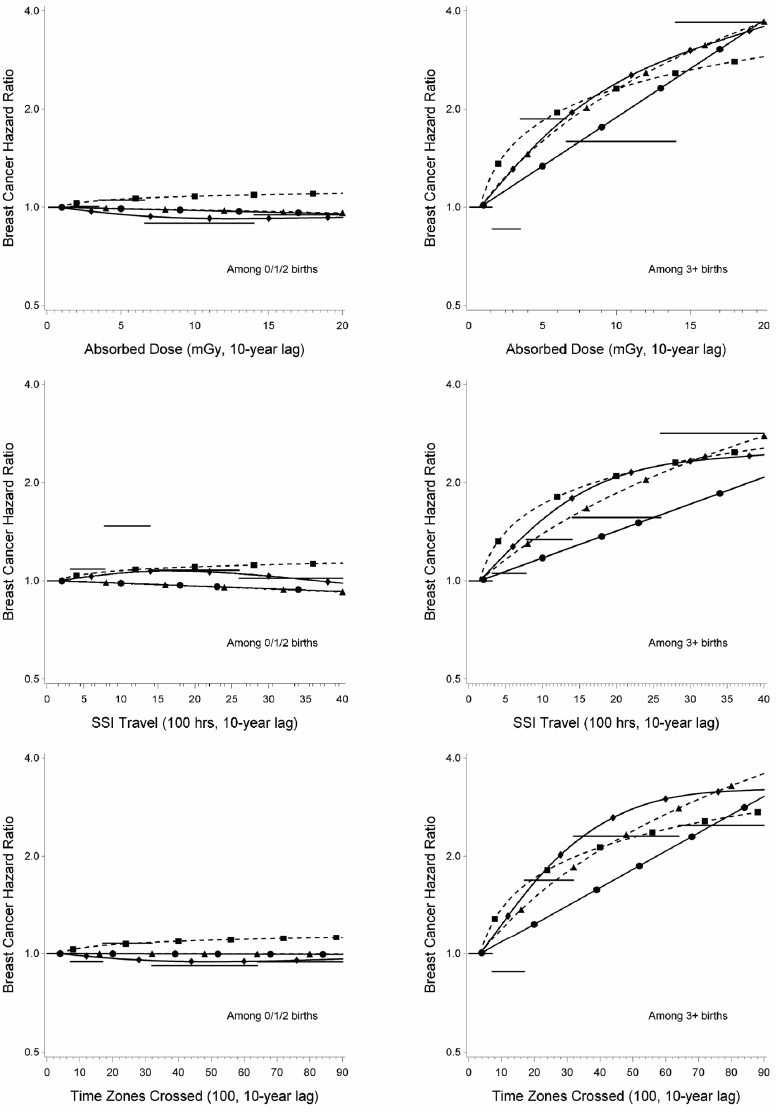
When the proportional hazards assumption was tested in the linear ERR models by including a three-way interaction term for exposure, parity, and age (<50 versus ≥50 years), the interaction terms were not statistically significant. However, the exposure effect among women with parity of ≥3 primarily occurred among women ≥50 years of age (supplementary table S4; results for women with parity <3 not shown, www.sjweh.fi/index.php?page=data-repository). Relatively few cases were observed among women with high parity at younger ages.
A post hoc evaluation of confounding indicated little confounding of the exposure estimates (<15% change in the exposure coefficient) by any of the covariates in the final models among cohort members with ≥3 births; exposure effect estimates among cohort members with fewer than three births were not statistically significant.
Discussion
The current study confirmed our previous findings of unexpected exposure–response relations only in women with high parity. Although based on a small subset (15%) of the cohort, these findings were observed in all model forms and persisted in all sensitivity analyses.
Other epidemiological data suggesting breast cancer incidence in flight attendants is associated with cosmic radiation dose or circadian rhythm disruption are limited. In the only other relatively large study evaluating both exposures (17), breast cancer risk was not associated with cosmic radiation dose after adjusting for parity or with number of flights crossing ≥6 time zones after adjusting for parity and age at first birth. None of the previous studies report evaluating effect modification by parity (17–23).
We could not assess the independent associations of cosmic radiation dose and circadian rhythm disruption with breast cancer incidence because the exposure metrics were highly correlated. Although model fits using the AIC criterion were similar for the power and linear ERR models, visually, the power model did not appear to fit well in the low exposure region among women with high parity, and overall the ERR models that are linear in the cumulative exposure D, in which relative risk = 1 + αD, appeared to be best-fitting. Previous studies have observed a linear relation between breast cancer risk and absorbed dose among other groups of radiation-exposed women (24). However, the ERR per 10 mGy observed in our study among the small subset of women with high parity is two orders of magnitude higher than predicted by the preferred model for breast cancer in BEIR VII (24). The predicted ERR per 10 mGy is 0.02 based on atomic bomb survivor data at an attained age of 50 years, the approximate mean attained age of women in the cohort with breast cancer. In addition, other data indicate that multiple births are associated with a reduction in the increased breast cancer risk associated with radiation exposure (24–26). The large difference between the observed and predicted ERR for women with high parity and the observed pattern of results by parity, which is the opposite of what would be expected for radiation-induced breast cancer, suggest that the observed exposure–response relations, if causal, are probably not due to cosmic radiation exposure but are more likely related to circadian disruption.
We previously speculated that women in our study with high parity may have been sleep-deprived from the home environment and therefore more sensitive to the effects of circadian rhythm disruption from work (4). A study that reported a linear increase in self-reported sleep insufficiency with the number of children in the home (27) provides some support for this speculation. In our study, women with high parity were more likely to have worked as a flight attendant after giving birth to a child than women with low parity. In addition, most women (87%) with high parity in the highest quartile of exposure for time zones crossed worked after giving birth to their third child.
Few data exist on effect modification by parity among workers who experience circadian rhythm disruption. In contrast to our study, effect modification by parity was not observed in a study of shift work and breast cancer among women in the Danish military (28). Effect modification by parity could be assessed in other studies of aircrew with data on parity and in other existing studies of workers who experience circadian rhythm disruption. Most studies of breast cancer incidence in flight attendants have little data on potential confounders beyond parity and age at first birth; however, our study suggests that this is not a major limitation.
Some of the observed associations of breast cancer risk with known breast cancer risk factors were unexpected. Former drinkers may have had a higher breast cancer risk than current drinkers because former drinkers drank more alcoholic drinks per week than current drinkers (data not shown). Although most women who used hormone replacement therapy did not have higher breast cancer risk than women who never took hormone replacement therapy according to the multivariable models, risk estimates for women with ≥3 years of use (lagged two years) were slightly higher and >1 in bivariate analyses (data not shown). The impact of hormone replacement therapy in the multivariable models may have been partially captured by menopausal status, which was based on whether the subject had a menstrual period in the last 12 months. We did not differentiate combined hormone replacement therapy from estrogen replacement therapy, and this may have also impacted our ability to detect a clear trend between breast cancer risk and duration of use. The unexpected results for age at first birth may be related to the observed occupational exposure effect modification by parity in conjunction with the correlation between parity and age at first birth. Breast cancer risk was not associated with body mass index in our study. However, the low mean body mass index and narrow distribution in the cohort, which reflect historically stringent height and weight requirements for employment as a flight attendant, limited our ability to detect an association.
Concluding remarks
Breast cancer incidence was not associated with estimates of cumulative cosmic radiation dose or measures of circadian rhythm disruption except among the small subset of women with high parity. If causal, the associations observed in high parity women seem more likely to be from circadian rhythm disruption than cosmic radiation. However, cautious interpretation of the findings in high parity women is warranted, and we recommend further study of breast cancer incidence among workers with circadian rhythm disruption to see if our findings are confirmed. Limitations of our previous analyses (low cumulative exposure, potential exposure misclassification, potential recall bias, relatively low participation) remain (4).