Urolithiasis is one of the most common urological diseases. Prevalence and incidence of urolithiasis have increased over the last decades (1). Several epidemiologic aspects have been proven to be related to the increasing likelihood of developing urinary stones, including genetics, age, sex, geography, seasonal factors, diet, and occupation (2). Many studies have indicated that there is a seasonal variation in the incidence of calculi (3–5). Temperature is especially considered to be a risk factor for urolithiasis.
Several studies suggested the linear correlation between climatic temperature and urolithiasis incidence. Geraghty et al (6) summarized these kind of studies in their systematic review (6). However, besides linear association, which was assessed using Pearson’s r, several studies have compared the incidence of urolithiasis at different climatic temperatures to show the effect using relative risk (RR) or odds ratio (OR). Studies have also suggested that heat exposure during the work can increase the risk of urolithiasis (7–9). Therefore, we conducted this systematic review and meta-analysis to assess comprehensively the effect of ambient temperature on the incidence of urolithiasis. In addition, many studies have used non-linear regression modelling to assess the association between climatic temperature and urolithiasis incidence, and these are also summarized in this review.
Methods
Literature search
We conducted a comprehensive literature search for studies published before 10 September 2019 in PubMed, Web of Science and Scopus according to the Preferred Reporting Items for Systematic Review and Meta-Analysis (PRISMA) statement using the search strategy: (climate OR weather OR season OR occupation OR work) AND (urolithiasis OR calculi OR nephrolithiasis OR renal colic OR kidney stone OR ureter stone OR bladder stone) AND (temperature OR heat OR hot). The exact search string is available in the supplementary material (www.sjweh.fi/show_abstract.php?abstract_id=3866, Document 1). The titles and abstracts of articles that were identified by the keyword search were screened against the study selection criteria. Potentially relevant articles were independently retrieved or evaluated for the full text. We also conducted a reference list and cited reference search from full-text articles that met the study criteria. Two independent reviewers screened all studies according to inclusion and exclusion criteria, and all disagreements were solved by discussion with a third author.
Study selection criteria
Studies that met the following criteria were included in this systematic review: (i) Participants: no specific requirements for participants, but the control group should be from the same population of the exposure group. (ii) Exposures: high climatic temperatures (with actual number above average local climatic temperature) or workplace with heat exposure. (iii) Comparator: normal or low climatic temperatures (with actual number not exceeding average local climatic temperature) or workplace without heat exposure. (iv) Outcomes: the events of urolithiasis, renal colic, or stone treatments were used as proxy of urolithiasis incidence. Outcomes contained at least one set of the following statistics: (a) duration of the study and Pearson correlation coefficient (Pearson’s r) of mean monthly temperature and incidence of urolithiasis; (b) RR with 95% confidence intervals (CI) of two different climatic temperatures or RR with 95% CI of comparison of workplace with or without heat exposure or they could be calculated from related data; (c) increased RR ratio (IRR) with 95% (CI) as mean daily temperature increased by a clear value. (v) Language: articles were written in English.
Data extraction and quality assessment
Two reviewers independently extracted data from every included study using a standardized form according to the PRISMA statement and evaluated all relevant clinical studies for methodological quality. The following information was extracted from each study: the name of the first author; year of publication; study location; study period; number of cases; Pearson’s r; RR for different ambient temperature; relative RR as mean daily temperature increased by a clear value (IRR). For convenience, the term RR (relative risk) will be used as a generic term for relative odds (cumulative incidence data), rate ratio (incidence-rate data) and OR (case–control data). Due to the lack of scales targeting time-series analysis study or case-crossover study, we created a scale – which combined several items from the New Castle-Ottawa Scale (www.ohri.ca/programs/clinical_epidemiology/oxford.asp) and the Cochrane risk of bias tool (10) – to assess the quality of these kinds of studies (supplementary Document 2). The quality of case–control study was evaluated using the New Castle–Ottawa Scale and the quality of cross-sectional study was evaluated using the Joanna Briggs Institution Checklist for Analytical Cross Sectional Study (joannabriggs.org/research/critical-appraisal-tools.html).
Data analysis
Pooled estimates of the Pearson’s r were calculated by transforming each r into Fisher’s Z value. The resulting value was then weighted with the inverse of the variance of the correlation coefficients. The 95% CI of the pooled weighted Fisher’s Z coefficients was also calculated, after which all of the values were back-transformed into r using the following formula (11):
To assess the non-linear association between increasing temperature and the incidence of urolithiasis, all IRR were standardized to an increase of 5 °C in climatic temperature. For studies reporting IRRu per U °C instead of standard level (eg, ten units), the IRRstandardised was calculated by the formula:
where U is the increment used in the original study to estimate the effects.
Potential publication bias was evaluated by using Egger’s linear regression test (with significance defined as P<0.05). Heterogeneity between studies was tested using both the I2 tests (I2<30% defined mild heterogeneity; 30%≤I2≤50%, moderate heterogeneity; and I2>50%, high heterogeneity). When heterogeneity was detected, the random effects model was adopted. If not, the fixed effects model was adopted (12). When results from fixed and random effects models were different from moderate heterogeneity (ie, I2=30–50%), we presented the latter as it represents a more conservative approach (13).
The pooled estimates of Fisher’s Z and IRR were conducted using RevMan 5.3 (the Nordic Cochrane Centre, Copenhagen, Denmark). The calculating of Egger’s linear regression test was conducted in STATA version 12.0 (Stata Corp, College Station, TX, USA). A subgroup analysis was planned beforehand to assess the effects of high climatic temperature and occupational heat exposure respectively. No sensitivity analysis was conducted.
Considering the heterogeneity of studies that evaluated the effect of climatic and workplace temperature using RR, a meta-analysis was not conducted. However, forest plots were still provided in order to present a visual representation of the direction and magnitude of these results.
Results
Characteristics of included studies
After removing duplicates, the initial database search yielded a total of 684 articles. After screening these titles and abstracts, 69 articles remained for full-text evaluation. Only 24 articles were eligible under the full-text assessment (figure 1). Of these, 9 studies directly investigated the impact of ambient temperature on urolithiasis using RR (7–9, 14–19), 12 time-series studies evaluated the linear correlation between mean monthly temperature and incidence of urolithiasis (5, 20–30), and 6 time-series studies estimated the increased RR as mean daily temperature increased to evaluate the non-linear association (28–33).
Tables 1–3 summarize the 24 studies that investigated the association between temperature and urolithiasis. Of these, 19 were time-series analyses, 4 were cross-sectional studies, and 1 was a case–control study. In terms of origin, 10 were from Asia, 5 from Europe, 6 from North America, 2 from Australia, and 1 was from South America. Emergency room (ER) visits were used in 15 studies to estimate the incidence of urolithiasis. Among these articles, 11 used ER presentation for renal colic, 4 demonstrated ER data for proven stones. Besides ER presentation, number of patients who sought medical evaluation and records of hospitalizations for proven stones were used respectively in 2 studies. The prevalence of calculi was used in 5 studies as a proxy for risk of urolithiasis. The records of extracorporeal shockwave lithotripsy (ESWL), emergency ambulance dispatches, and the number of stones submitted to the biochemistry department to evaluate the stone formation were each used in 1 study, respectively.
Table 1
Characteristics of studies that assessed the effect of ambient temperature. [CS=cross-sectional study; CC=case-control study; TS =time-series study; RR=relative risk].
Study | Country | Study period | Risk parameters | Cases (N) | Study type | RR (95% CI) | Exposure and dose | Confounders |
---|---|---|---|---|---|---|---|---|
Lee et al, 2016 (14) | Korea | 2005–2013 | Patients visiting emergency department as proven stones | 14 518 | TS | 2.54 (1.67–3.87)a | Daily mean temperature 29 0C versus 13 0C | Humidity, sea level, pressure, sunshine |
Ordon et al, 2016 (15) | Canada | 2002–2013 | Patients visiting emergency department for renal colic | 423 396 | TS | 1.30 (1.20–1.42)a | Daily mean temperature 21.1 0C versus –7.0 0C | Age, sex, income, residence region, basic diseases |
Safarinejad et al, 2007 (17) | Iran | 2005 | Prevalence obtained from 30 counties of Iran using questionnaire | 436 | CS | 1.9 (1.7–2.2)a | Mean annual temperature >26 0C versus <15 0C | Obesity, hypertension, unemployment, consumption of liquid, cola, meat and coffee |
Tasian et al, 2014 (18) | USA | 2005–2011 | Patients who sought medical evaluation or treatment of kidney stones in 5 cities | 60 433 | TS | 1.38 (1.07–1.79)a | Daily temperature 30 0C versus 10 0C in Atlanta | Relative humidity |
1.37 (1.07–1.76)a | Daily temperature 30 0C versus 10 0C in Chicago | |||||||
1.36 (1.10–1.69)a | Daily temperature 30 0C versus 10 0C in Dallas | |||||||
1.11 (0.73, 1.68)a | Daily temperature 30 0C versus 10 0C in Los Angeles | |||||||
1.47 (1.00–2.17)a | Daily temperature 30 0C versus 10 0C in Philadelphia | |||||||
Yang et al, 2016 (19) | China | 2008–2012 | Daily ambulance dis–patches for renal colic from emergency center | 3158 | TS | 1.92 (1.21–3.05)a | Daily temperature 30.7 0C versus 21 0C | Humidity |
Atan et al, 2005 (7) | Brazil | 1999–2002 | Prevalence in male workers at a large steel plant | 181 | CS | 9.98 (7.39–13.47)b | Hot work area (>45 0C) versus non–hot work area (room temperature) | |
Borghi et al, 1993 (8) | Italy | unclear | Prevalence in male machinists at a large glass plant | 24 | CS | 3.73 (1.25–11.12)b | Hot work area (29–31 0C) versus non–hot work area (24–27 0C) | |
Luo et al, 2014 (9) | China | 2003–2010 | Prevalence in workers at a shipbuilding company | 190 | CC | 6.1 (3.4–10.9)b | Welder versus Indoor | |
3.6 (2.0–6.6)b | Assembler versus Indoor | |||||||
3.5 (1.9–6.3)b | PSQI (Production security and quality inspector) versus indoor | |||||||
6.4 (2.9–13.9)b | Smelter worker versus indoor | |||||||
7.2 (1.9–27.4) | Planing machine operator versus indoor | |||||||
6.9 (2.7–17.7)b | Spray painter versus indoor | |||||||
4.3 (1.3–14.6)b | Gas–cutting worker versus indoor | |||||||
Pin et al,1992 (16) | Singapore | unclear | Prevalence in male workers in several occupations | 16 | CS | 5.53 (0.72–42.77)b | Outdoor versus indoor |
Table 2
Characteristics of studies that assessed linear correlation using Pearson’s r of monthly climatic temperature and incidence of urolithiasis. [RC=emergency room (ER) visits for renal colic; SS=stones submitted to the biochemistry department; ESWL=records of extracorporeal shockwave lithotripsy; PS=ER visits for proven stones; NS=Not specified].
Study | Study period and number of months | Country | Case type | Data source | Cases (N) | Pearson’s r | Confounders |
---|---|---|---|---|---|---|---|
al-Hadramy et al, 1997 (5) | 1992–1994 (N=36) | Saudi Arabia | RC | 1 hospital | 1342a | 0.67 | Air pressure, humidity |
Buttigieg et al, 2016 (20) | 2009–2011 (N=36) | Malta | SS | 1 hospital | 389 | 0.49 | Humidex, humidity |
Cepeda et al, 2015 (21) | 2005–2013 (N=108) | Spain | RC | 1 hospital | 9330 | 0.336 | Humidity, sunshine |
Chauhan et al, 2004 (22) | 1996–2002 (N=84) | USA | RC | 15 New Jersey ER | 30 358 | 0.42 | NS |
Chen et al, 2008 (23) | 1999–2003 (N=60) | Taiwan | RC | All patients in Taiwan | 270 302 | 0.517 | Air pressure, sunshine |
Condemi et al, 2015 (28) | 2007–2010 (N=48) | Italy | RC | 1 hospital | 4051 | 0.60 | Age, air pressure |
Gaziev et al, 2016 (24) | 2007–2009 (N=35) | Italy | RC | 1 hospital | 2682 | 0.611 | Air pressure, humidity |
Lin et al, 2014 (25) | 2006–2011 (N=60) | Taiwan | ESWL | 1 hospital | 6616 | 0.696 | Humidity, rainfall, air pressure, sun, wind |
Lo et al, 2010 (29) | 1999–2007 (N=108) | New Zealand | RC | All public hospitals in Auckland | 7668 | 0.40 | Sunshine |
Park et al, 2015 (30) | 2006–2010 (N=60) | Korea | RC | Nationwide data | 1 702 913 | 0.557 | Humidity, sunshine, air pressure |
Sirohi et al, 2014 (26) | 2007–2012 (N=72) | USA | PS | 1 hospital | 3647 | 0.3995 | Humidity, rainfall, air pressure |
Freeg et al, 2012 (27) | 2007–2009 (N=36) | UAE | PS | 1 hospital | 458 | 0.079 | Humidity |
Table 3
Characteristics of studies that evaluated non-linear association using increased relative risk (IRR). [ER=emergency room.]
Source author | Study period | Country | Case type | Data source | Cases (N) | IRR and 95%CI |
---|---|---|---|---|---|---|
Borg et al, 2017 (31) | 2003–2014 | Australia | ER admissions for renal colic | All metropolitan hospitals in Adelaide | 19171 | 1.016 (1.011–1.020)/ 1 0C |
Condemi et al, 2015 (28) | 2007–2010 | Italy | ER visits for renal colic | S. Croce and Carle Hospital of Cuneo | 4051 | 1.11 (1.03–1.19)/ 5 0C |
Fletcher et al, 2012 (32) | 1991–2004 | USA | Acute-care hospital admissions as renal calculi | New York State | 34 087 | 1.06 (1.05–1.08)/ 5 0C (2.78 0C) |
Acute-care hospital admissions as lower urinary calculi | New York State | 2205 | 1.09 (1.04–1.16)/ 5 0C (2.78 0C) | |||
Lo et al, 2010 (29) | 1999–2007 | New Zealand | ER visits for renal colic | All public hospitals in Auckland | 7668 | 1.028 (1.013–1.043)/ 1 0C |
Park et al, 2015 (30) | 2006–2010 | Korea | ER visits for renal colic | Nationwide data | 1 702 913 | 1.0171 (1.0102–1.0241)/ 1 0C |
Malig et al, 2019 (33) | 1999–2009 | USA | Records of hospitalizations due to kidney stone | Statewide data of California | NS | 1.152 (1.103–1.204) / 10 0C (5.56 0C) |
In the quality assessment of time-series analyses, 7 studies were considered to be of good quality, 11 intermediate quality, and 1 of low quality (supplementary table S2). Supplementary table S3 summarizes the quality of cross-sectional studies with one considered to be of low quality. The case–control study was considered to be good quality using the Newcastle–Ottawa Scale (supplemental table S4).
Effect of ambient temperature on urolithiasis risk assessed with relative risk
RR information was complete in 9 studies and these were included in the systematic review. The overall effects of ambient temperature on urolithiasis risk were not calculated due to the heterogeneity of exposure measurement. The effect of climatic temperature (figure 2A) was assessed in 5 studies: 4 compared the daily urolithiasis events of different temperature (14, 15, 18, 19). Most studies reported significantly different urolithiasis risks, with two temperatures differing as much as 10 °C in Yang et al’s study (19) to 28 °C in Ordon et al’s study (15). However, Tasian et al reported data from five US metropolitan areas, and the RR of urolithiasis cases in Los Angeles did not reach statistical between temperatuers of 10–30 °C (18). In fact, the RR stopped rising after 15 °C, and considering the relatively lower urolithiasis incidence compared to other cities (167 kidney stone incidence per 100 000 in Los Angeles and 256 per 100 000 in Philadelphia, which is the lowest of the other four cities), the Mediterranean climate of Los Angeles and other socioeconomic factors might be the reason for the insignificance of the RR. In their analysis of the average annual temperatures reported, Safarinejad et al (17) concluded that higher annual temperature levels were related to more urolithiasis events.
Figure 2
The forest plot of the effect of ambient temperature on incidence of urolithiasis. The overall effects were not calculated because of the heterogeneity of exposure measurement, and forest plots were provided for visual representation of outcomes. [SE=standard error; CI=confidence interval]. (A) The effect of climatic temperature on urolithiasis incidence; (B) the effect of heat exposure during work on urolithiasis incidence.
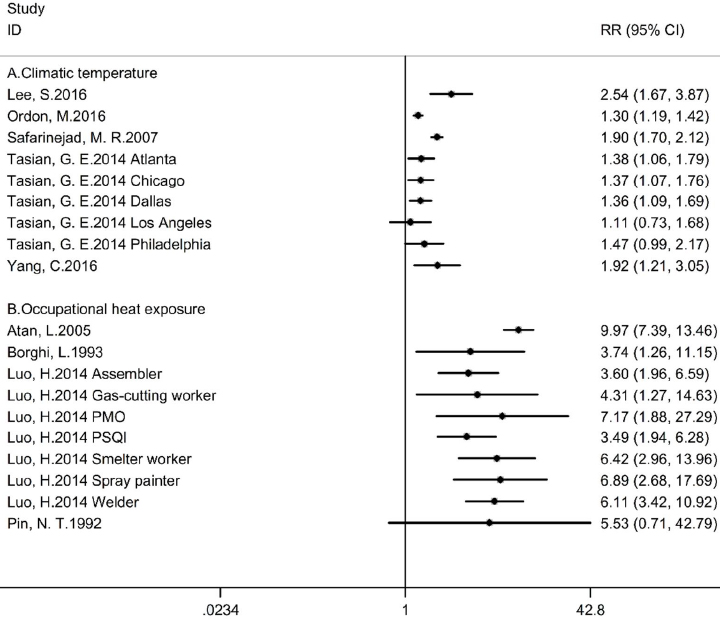
The effect of occupational heat exposure was investigated in 4 studies (figure 2B). Pin et al (16) compared the prevalence of stone diseases of five occupations and found that urolithiasis occurred more among outdoor workers (15 of 288) than indoor workers (1 of 118), though this was not statistically significant (16). Borghi et al (8) then compared the calculi prevalence of workers exposed to hot temperatures (Wet Bulb Globe Temperature, WBGT: 29–31 °C) against those of workers in normal temperature (WBGT: 24–27 °C) at a glass plant in Italy, and significant differences were found (20 of 236 in hot temperature versus 4 of 165 in normal temperature). Another study conducted at a steel industry in Brazil by Atan et al (7) also supported the effect of heat exposure, in which the hot-area workers (103 of 1289) had a significantly higher prevalence of urolithiasis than those who worked in areas at room temperature (78 of 9037). In their research that focused on workers in a shipbuilding company in China, Luo at al (9) also reported relatively higher urolithiasis risk among the outdoor workers.
It should be noted that the studies that assessed the effect of temperature using RR were quite heterogeneous in study design, and the quality assessment of these studies were based on three different instruments. Therefore, these conclusions should be interpreted with caution.
Linear association of climatic temperature and urolithiasis risk
To pool the linear association between monthly temperature and urolithiasis risk, 11 studies containing Pearson’s r were included. The Fisher’s Z was 0.53 (95% CI 0.41–0.65) and the summary r was 0.49 (95% CI 0.40–0.57) (figure 3), which means a moderate association (0.4<r<0.6).
Figure 3
The forest plot of the Pearson’s r of monthly mean temperature and incidence of urolithiasis. [SE=standard error; CI=confidence interval].
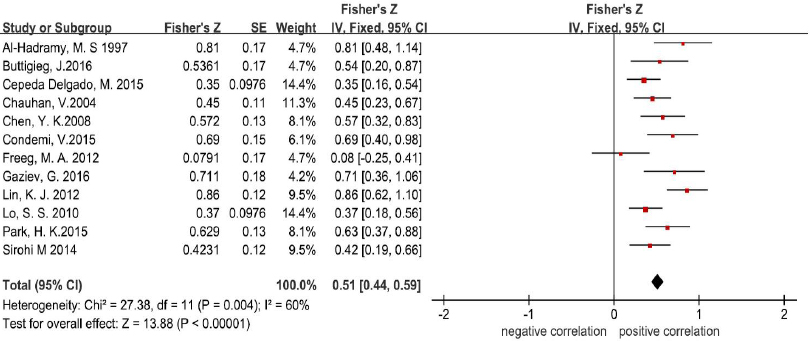
Related statistics of three regions were reported in 2 studies. After pooling the related outcomes, we found that in the USA, the association was weak to moderate (r=0.41, 95% CI 0.27–0.54), while in Italy and Taiwan, the association was moderate to strong (r=0.60, 95% CI 0.44–0.73; r=0.62, 95% CI 0.41–0.76). Then we performed a subgroup analysis based on the countries. In Europe (Malta, Spain, and Italy), the association was weak to moderate (r=0.49, 95% CI 0.34–0.62), and in East Asia (Taiwan and Korea) a moderate-to-strong association was suggested (r=0.60, 95% CI 0.48–0.70). Notably, though Saudi Arabia and United Arab Emirates are neighboring countries, the reported association between temperature and incidence of urolithiasis was vastly different, and the economic conditions and lifestyles might be the sources of difference.
The non-linear association of climatic temperature and urolithiasis risk
In addition to studies that assessed the linear association between climatic temperature and urolithiasis risk, many were assessed non-linear associations, 6 of which were included in our analysis (figure 4) (28–33). All these studies consistently reported that the urolithiasis risk increased with the daily temperature. Our findings show that when the daily increased by 5 °C, the urolithiasis risk increased by 10% (95% CI 8–11%).
Publication biases
The publication bias of the analysis of Pearson’s r using Egger’s test was not significant (P=0.279). The funnel plot is shown in figure 5A, and two studies suggested biases. After excluding the biased studies, the outcomes remained consistent with the previous results (Fisher’s Z: 0.50; 95% CI 0.42–0.58). The results of the non-linear association analysis showed no publication bias (Egger’s test: P=0.095; figure 5B).
Figure 5
(A) The funnel plot of the linear association between monthly mean temperature and incidence of urolithiasis. (B) The funnel plot of the non-linear association between daily mean temperature and incidence of urolithiasis. The risk ratios was standardized to increased risk ratio for per 5°C increment. [SE=standard error; CI=confidence interval.]
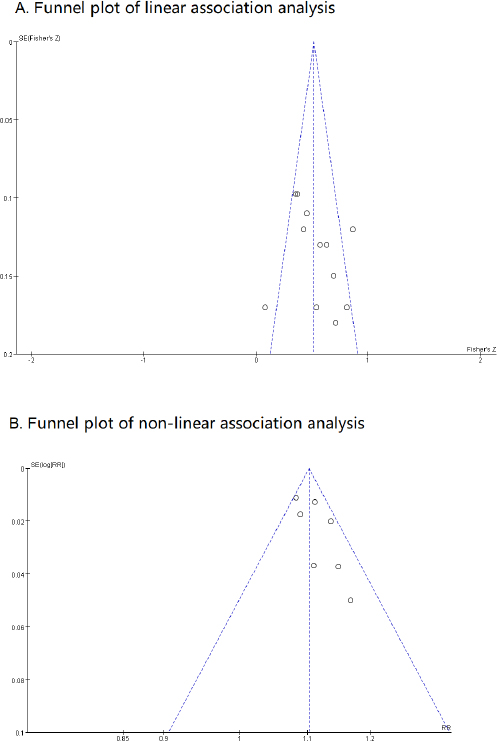
Discussion
The present systematic review and meta-analysis analyzed 24 studies and demonstrated a significant positive association between ambient temperature and risk of urolithiasis. Our study suggested that the increased temperature in climate and workplace could significantly increase urolithiasis incidence.
To assess the linear association between climatic temperature and urolithiasis risk, Pearson’s r of mean monthly temperature was extracted. However, in addition to mean monthly temperature, other temperature parameters may be related to the incidence of urolithiasis. Both Cepeda Delgado’s and Lin’s studies revealed that the monthly maximum, average, and minimum temperature were all linearly related to the risk of urolithiasis (21, 25, 34). Daily data were also used in several studies, and Cervellin et al found a significant association between the mean number of cases of renal colic per day and the mean daily temperature (positive association, r=0.93; P<0.0001) (35, 36). In another study, the daily maximum, average, and minimum temperature were all significantly related to the incidence of urolithiasis (37). Furthermore, two articles suggested an association between annual mean temperature and urolithiasis (17, 38). It has been proposed that global warming will lead to an increase of 1.6–2.2 million lifetime cases of urolithiasis by 2050 in US, which might be as high as 30% in some areas (39). These articles support our conclusion and the need for greater action on global warming to decrease the risk of urolithiasis. However, more research is needed as there are still some studies that do not support the relationship between temperature and urolithiasis (27, 40). We excluded Power’s study (40) from the meta-analysis, but the mild climatic of Britain could be a reason for the insignificant result.
Regarding the studies that compared different temperature using RR, we did not conduct a meta-analysis to calculate the overall effect due to heterogeneity as these studies comprised different designs, and both prevalence and incidence were used. The dose of exposure was also a source of heterogeneity. For studies investigating climatic temperature, the normal climatic temperature of different districts was diverse, and the temperature used for comparison also varied in these studies. For studies focusing on workplace temperature, the measurement of temperature was hard and the heat exposure of different types of work also differed.
There was a difference between the effect of climatic and workplace temperatures on the incidence of urolithiasis (figure 2). One probable reason is that the heat exposure in work can reach as much as 50 °C, which is much higher than normal climatic temperature (7). Moreover, when the climatic temperature increases, people tend to stay indoors with air conditioning, which may reduce the effect. Another explanation is that heat exposure at work is consistent for years, and the heavy physical burden could have aggravated the effect. Apart from these reasons, the quality and quantity of studies included may have an effect on these outcomes. The data collected for heat exposure at work were crude (ie, without adjustment for confounders) and may have resulted in an overestimation of the effect. For example, in the study that compared several outdoor occupations, the OR of planning machine operator (unadjusted: P<0.01; adjusted: P=0.06) and gas-cutting worker (unadjusted: P=0.02; adjusted: P=0.15) were insignificant after adjustment for exposure time and blood pressure. But adjusted OR of other occupations and the overall difference between outdoor and indoor workers remained significant.
We had a theory on the mechanisms behind the relation of temperature and incidence of urolithiasis. Heat exposure can result in sweating, leading to decreased extracellular fluid (ECF) and subsequent dehydration (41). Low ECF leads to increased secretion of vasopressin, which promotes reabsorption of water from the renal filtrate and increases urinary concentration (42, 43). The increased urinary calcium and super-saturation of calcium oxalate and calcium phosphate may significantly increase the risk of calculi. The relatively lower RR in Ordon’s study may support this theory because the climatic temperature of this Canadian study is obviously lower than in other studies and, therefore, the dehydration might not significantly increase at 21 °C (15). The insignificant outcome of the study conducted in Britain may also support this (40).
To our knowledge, the present study is the first meta-analysis to estimate the effect of temperature on the incidence of urolithiasis. This review is based on worldwide data from 14 different countries involving over 2.5 million cases. We evaluated the linear correlation between temperature and urolithiasis by pooling Pearson’s r using data over 707 months. We further estimated the non-linear correlation that the risk of urolithiasis increased by 10% (95% CI 8–11%) with each 5 °C increment in temperature.
This study had some limitations, including low evidence level of included studies, potential improper assessment of study quality, and potential misclassification of heat exposure. Most of the included studies were time-series analyses, which do not strongly indicate causal relationships. We adopted the standard to assess the quality of time-series analysis from authoritative scales, but it has not been well tested. Therefore, we tried not to overestimate quality. We were unable to measure heat exposure accurately in these studies because the coverage of each study is vast and the climatic temperature varies inside the district. Hence, we added the matching degree of climate and district to the quality assessment. In addition, because of the resource restraints, we only included English articles, but several studies in Russia, Spanish and other languages might have contained relevant outcomes. Lastly, confounders such as drinking habits and physical burden were not well controlled for in original outcomes, and we are unlikely to have eliminated all biases.
Concluding remarks
In summary, there is an association between temperature and incidence of urolithiasis. The high temperature of both climate and workplace environments are related to increased urolithiasis risk. Public health intervention strategies need to be developed to prevent those operating in high-risk areas or occupations from being exposed to high ambient temperature.