Carpal tunnel syndrome (CTS), determined by the compression of the median nerve within the carpal canal at the wrist, is broadly recognized as the most common mononeuropathy (1). It may adversely affect the quality of life of the patient (sleep disruption as the symptoms – tingling, burning and pain in the first three fingers of the hand – typically present during the night, and/or loss of sensitivity and force, which may affect manual dexterity) (2). CTS incidence in the general population has been reported to be as high as 3 cases per 1000 person-years, and CTS surgery is very common as well (1 per 1000 person-years) (3, 4). Surgically-treated CTS was three to seven times more common (depending on age/gender) among manual compared to non-manual workers (5). Thus, CTS is a common cause of work disability and determines high healthcare expenditures (6, 7). A large amount of literature documented that CTS is associated with several personal characteristics, including increasing age, overweight/obesity, female gender, and musculoskeletal comorbidities (eg, trigger finger, rotator cuff syndrome, and cervical radiculopathy – double crush syndrome) (8–12). Many clinical investigations have focused on the role of systemic diseases in increasing the risk of CTS. However, the proportion of cases attributable to these factors is probably limited as they are rare (eg, systemic amyloidosis, sarcoidosis) or only weakly associated with CTS (eg, diabetes, hypothyroidism) (13–16). During the eighties, a landmark study posited an association between fast and forceful manual work and CTS (17): since then, the issue of occupationally induced CTS has been the subject of a number of epidemiological studies, although the majority of them were cross-sectional in design (18). Recently, two large longitudinal studies on CTS and fast and forceful manual work were published, from Italy (19) and the USA (20). To date, these are the largest and most informative studies published on the issue, mainly because of the design, the number of subjects observed, the rigorous case definition used, and the detailed assessment of the exposure.
Here, we report the last follow-up of the Italian cohort (the OCTOPUS study), presenting data on industrial/service workers followed-up for up to ten years. In this setting, representing the largest prospective cohort study ever published, we aimed at investigating the role of ergonomic and personal risk factors in the genesis of CTS, as well as their relative contribution to the burden of the disease.
Methods
The OCTOPUS study has been described in detail elsewhere (19, 21): here we briefly summarize the main features of the study population and methods used for exposure assessment, outcome assessment and statistical analyses.
Study population
The OCTOPUS cohort was established in 2000–2001 (first examination): workers enrolled in the study were full-time employees of seven industrial (tiles, small appliance, large appliances, garment and shoes – two companies – manufacturing) and service (nursery and early childhood centers) organizations. At each subsequent examination (2001–2002, 2002–2003, 2008–2011) newly hired workers in each company where invited to participate in the study (open cohort design). Four companies (tiles, small appliance, large appliances, and one of the shoes manufacturing) participated to all follow-up assessments. One of the shoes industries and the nursery and early childhood centers voluntary exited the study after the third follow-up assessment. The garment manufactory closed down after the second follow-up assessment.
More details about the number of workers enrolled in the study are reported in figure 1.
Exposure assessment
Exposure to fast and forceful manual work was assessed according to the American Conference of Governmental Industrial Hygienists (ACGIH) threshold limit value (TLV)® method (22) by a team of trained professionals (ergonomists and industrial hygienists) who rated all suitable jobs in each company. Assessment was performed at task level, based mainly on observation (with videotapes whenever possible) and was complemented, where available, by standard production times and data. Many tasks involved the use of both hands and the exposure level of each hand was assessed. For each task, the ergonomists evaluated the exposure level of the hand-wrist system in terms of hand activity level (HAL) and normalized peak force (nPF). Then, we linked each worker to the estimated exposure values based on the assigned tasks. In presence of job rotation, we assigned to each worker the average value of HAL calculated over all performed jobs and the highest PF estimated for the pool of jobs under consideration. Finally, we combined HAL and nPF values using the formula nPF/(10-HAL) and we compared the estimated value against the action limit (AL) and the TLV proposed by the ACGIH (22). Exposure to hand–arm vibrations (HAV) was assessed in terms of presence or absence; workers performing jobs in which any vibratory tools were used for any work-cycle, independently of total time of usage, were considered exposed to HAV.
Outcome assessment
Each worker underwent a clinical examination by a trained physician to collect symptoms of CTS and other personal variables using a structured questionnaire. During the first three follow-up assessments, each worker who was symptomatic for CTS underwent median nerve conduction study of both hands, according to a standard method (21). During the last follow-up assessment (2008–2011), all the subjects underwent nerve conduction studies (NCS), irrespective of the presence of symptoms. Physicians performing clinical assessment were unaware of exposure data collected for the company and NCS were performed without knowledge of the clinical data of the subject.
We used two different case definitions: (i) presence of CTS symptoms in the 30 days before the interview (hereinafter referred to as “CTS symptoms”); and (ii) presence of CTS symptoms and slowing of sensory conduction velocity of the median nerve from wrist to palm (referred to as “CTS confirmed by NCS”). Symptoms were classified according to the consensus criteria for the classification of CTS in epidemiologic studies (23). Information was collected with a structured questionnaire including the Katz hand diagram (24); subjects who reported numbness, tingling, burning, or pain in one or more of first three fingers of the hand (“possible” or “classic/probable” symptoms of CTS) were classified as symptomatic cases. We also performed a sensitivity analysis based only on subjects who reported classic/probable symptoms.
Experienced electro-diagnostic technicians performed NCS, as described elsewhere (21). The short-segment (8 cm) sensory conduction velocity of the median nerve from wrist to palm was classified as “slowed” if it fell below the lower 99% confidence limit (43.8 m/s) of the electro-diagnostic reference values described by Kimura (25). The Institutional Review Board of the University Hospital of Bologna approved the study protocol (n. 054/2000/O).
Statistical analysis
Only subjects with complete information on selected covariates entered the main analyses (list wise deletion). Summary statistics were expressed as numbers (percentages), means [standard deviation (SD)], or medians [interquartile range (IQR)] as appropriate. The correlation between variables was studied using Spearman’s rank correlation coefficient (Spearman’s rho).
We fitted Cox proportional hazards regression models with robust standard errors clustered on the seven companies to estimate hazard ratios (HR) and relative 95% confidence intervals (95% CI). Covariates to be included in the multivariable models were selected with a “significance-test-of-the-covariate” strategy. We started from the full model, which included all the pre-selected baseline covariates alongside the variable for the ACGIH TLV® method; then, we performed a backward deletion, retaining the variables that reached the statistical significance level of P<0.1 at the likelihood ratio test. After preliminary analysis, gender, age, body mass index (BMI), and presence of pathologies predisposing to CTS onset were retained as covariates in multivariate models. Of note, exposure to HAV did not reach the predefined significance level for the selection of covariates (P<0.1) for any of the outcome variables. Age, ACGIH TLV® categories, HAL, and nPF were treated as time-varying covariates; for each person-year, the exposure was classified independently. To assess the presence of linear trends across levels of ordinal variables, we evaluated the Wald chi-square statistic after fitting regression models including a linear term for the covariate of interest.
Population attributable fractions (PAF), defined as the percentage of cases attributable to the exposure of interest, were estimated after fitting regression models through maximum likelihood methods (26). To give a perspective on the role of the studied risk factors in the genesis of each observed CTS case, we applied the method proposed by McElduff and colleagues to estimate the proportional contribution of multiple risk factors to the development of a disease in an individual (27). This method allows partitioning the excess risk so that the contribution of each risk factor plus an additional component for “unknown” risk factors sum up to 100%. The proportional contribution may be interpreted as a lower bound value for the probability of causation (27).
All the analyses were performed using Stata 14.1 SE (Stata Corp, College Station, TX, US). All the tests were two-sided and we defined as statistically significant a P-value <0.05.
Results
Panel A of figure 1 reports the flow diagram of the study population. Out of the 4734 invited workers, there were 4505 workers eligible for the cohort: of these, 273 (6%) were non-responders. As shown by Panel B of figure 1, four outcome assessments were performed in this dynamic cohort. A total of 1101 subjects were lost to follow-up after their baseline assessment; 693 of them had participated only to the first cohort assessment, 231 to the second, and 177 to the third one. The dynamic cohort includes 860 workers entered after the first follow-up assessment and 825 subjects were still under follow-up at the end of the study. The dropout rates due to personal factors (ie, subjects who quitted the study while their company was still under follow-up) were: 16.4% after the first follow-up assessment; 16.5% after the second follow-up assessment; 20.3% after the third follow-up assessment. Also, 191 workers (4.5%) left the study after the second follow-up assessment and 967 (22.8%) after the third one because their companies abandoned the study.
All subjects symptomatic at their baseline or during follow-up (N=882) were invited to undergo NCS: 245 (28%) of them refused to undergo the tests (of these, 122 were classified as incident cases according to the definition based on solely symptoms). Supplemental table A (www.sjweh.fi/data_repository.php) presents a summary of baseline characteristics by participation to NCS: we observed minor differences only for the distribution of gender and ACGIH TLV® categories.
When investigating the incidence of CTS symptoms, we excluded 451 prevalent cases at their baseline and we analyzed data on 431 incident cases in 8000 person-years with an overall incidence rate of 5.4 per 100 person-years. Of these, 119 cases (28%) and 3286 person-years (41%) represented the incremental contribution of the forth follow-up assessment. When investigating CTS confirmed by NCS, after the exclusion of 108 prevalent cases, we observed 126 incident cases in 8883 person-years (overall incidence rate, 1.4 per 100 person-years). The fourth follow-up assessment accounted for 55 cases (44%) and 3813 person-years (43%). Incidence rates (per 100 person-years) of CTS symptoms in the studied population by ACGIH TLV® method and personal characteristics are presented in table 1. As expected, we documented higher rates among females and with increasing age and BMI. Remarkably, no linear trend across ACGIH TLV® categories was observed; indeed, subjects in the intermediate category (above AL and below TLV) presented higher rates compared to those in the upper category (above the TLV). Incidence rates of CTS confirmed with NCS are presented in supplemental table B (www.sjweh.fi/data_repository.php).
Table 1
Incidence rates (per 100 person-years) of CTS symptoms in the studied population by ACGIH TLV® method and personal characteristics. The OCTOPUS cohort, Italy, 2000–2011. [ACGIH=American Conference of Government Industrial Hygienists; AL=action limit; CTS=carpal tunnel syndrome; IR=incidence rate; Pyrs=person-years; TLV=threshold limit value; 95% CI=95% confidence intervals].
Biomechanical exposure classified according to ACGIH TLV® method | ||||||||||||
---|---|---|---|---|---|---|---|---|---|---|---|---|
|
||||||||||||
Below AL | Between AL and TLV | Above TLV | ||||||||||
|
|
|
||||||||||
Cases | Pyrs | IR | 95% CI | Cases | Pyrs | IR | 95% CI | Cases | Pyrs | IR | 95%CI | |
Entire cohort | 187 | 4946.0 | 3.8 | 3.3–4.4 | 107 | 1080.5 | 9.9 | 8.2–12.0 | 137 | 1973.5 | 6.9 | 5.9–8.2 |
Sex | ||||||||||||
Male | 55 | 2692.0 | 2.0 | 1.6–2.7 | 33 | 402.5 | 8.2 | 5.8–11.5 | 28 | 822.0 | 3.4 | 2.4–4.9 |
Female | 132 | 2254.0 | 5.9 | 4.9–6.9 | 74 | 678.0 | 10.9 | 8.7–13.7 | 109 | 1151.5 | 9.5 | 7.8–11.4 |
Age (years) | ||||||||||||
Up to 35 | 41 | 2194.5 | 1.9 | 1.4–2.5 | 34 | 483.5 | 7.8 | 5.5–10.9 | 42 | 959.0 | 4.4 | 3.2–5.9 |
36–45 | 81 | 1758.5 | 4.6 | 3.7–5.7 | 42 | 410.0 | 10.2 | 7.6–13.9 | 47 | 584.5 | 8.0 | 6.0–10.7 |
46–55 | 59 | 925.0 | 6.4 | 4.9–8.2 | 28 | 224.0 | 12.5 | 8.6–18.1 | 41 | 409.0 | 10.0 | 7.4–13.6 |
>55 | 6 | 68.0 | 8.8 | 4.0–19.6 | 3 | 8.0 | 37.5 | 12.1–116 | 7 | 21.0 | 33.3 | 15.9–70 |
Body mass index (kg/m2) | ||||||||||||
<25 | 115 | 3363.5 | 3.4 | 2.8–4.1 | 70 | 751.5 | 9.3 | 7.4–11.8 | 76 | 1335.0 | 5.7 | 4.5–7.1 |
25.0–29.9 | 53 | 1234.5 | 4.3 | 3.3–5.6 | 26 | 251.0 | 10.4 | 7.1–15.2 | 48 | 510.5 | 9.4 | 7.1–12.5 |
≥30.0 | 19 | 348.0 | 5.5 | 3.5–8.6 | 11 | 78.0 | 14.1 | 7.8–25.5 | 13 | 128.0 | 10.2 | 5.9–17.5 |
Predisposing diseasesa | ||||||||||||
0 | 163 | 4717.5 | 3.5 | 3.0–4.0 | 99 | 1030.5 | 9.6 | 7.9–11.7 | 116 | 1864.0 | 6.2 | 5.2–7.5 |
≥1 | 24 | 228.5 | 10.5 | 7.0–15.7 | 8 | 50 | 16.0 | 8.0–32.0 | 21 | 109.5 | 19.2 | 12.5–29.4 |
Among the 3131 subjects with complete information at their baseline, the mean age was 37.9 (SD 9.5) years, the mean BMI was 24.0 (SD 4.0) kg/m2, and median current job seniority was 7 (IQR 2–15) years. The cohort consisted of 2032 (65%) women [mean age 39.3 (SD 9.4) years, mean BMI of 23.4 (SD 4.1) kg/m2, and median current job seniority of 8 (IQR 2–18) years] and 1099 (35%) men [mean age 35.3 (SD 9.1) years, mean BMI of 25.0 (SD 3.4) kg/m2, and median current job seniority of 6 (IQR 2–11) years]. Baseline characteristics according to follow-up status are presented in supplemental table C (www.sjweh.fi/data_repository.php).
The distribution of the study population according to HAL, nPF and gender is presented in supplemental figure A (www.sjweh.fi/data_repository.php). Overall, a moderate correlation (Spearman’s rho 0.42) was found between the values of HAL and nPF at the baseline; the correlation was stronger among women (Spearman’s rho 0.51) than men (Spearman’s rho 0.28).
Table 2 presents the estimates of the multivariate Cox regression models selected to study the relationship between ACGIH TLV® categories and risk of CTS. The HR of CTS symptoms and CTS confirmed by NCS showed a similar pattern in relation to the category of ACGIH TLV®: compared to subjects exposed below the AL, both workers with exposure levels between the AL and the TLV or above the TLV showed a two-fold increase in risk. The magnitude of the risks associated with personal characteristics varied depending on the case definition. Advanced age was the most important predictor for both case definitions, but the HR estimated when investigating CTS confirmed by NCS were almost two times higher than those observed for CTS symptoms. Similarly, BMI was strongly associated with CTS confirmed by NCS, but not with CTS symptoms. On the opposite, estimates for gender and presence of predisposing diseases were similar when investigating CTS symptoms or CTS confirmed by NCS. Supplemental table D (www.sjweh.fi/data_repository.php) presents the estimates obtained when considering a more stringent definition for symptoms (only subjects reporting “classic/probable” symptoms). The change of the case definition determined only a minor loss of cases [60 (13.9%) for CTS symptoms and 9 (7.1%) for CTS confirmed by NCS]. All the estimates obtained from this sensitivity analysis were in line with those obtained when including in the case definition both “classic/probable” and “possible” symptoms.
Table 2
Results from Multivariate Proportional Hazards Regression Models including exposure classified according to American Conference of Government Industrial Hygienists (ACGIH) threshold limit value (TLV)® method alongside selected covariates. The OCTOPUS cohort, Italy, 2000–2011. [AL=action limit; CTS=carpal tunnel syndrome; HR=hazard ratio; NCS=nerve conduction studies; Ref=reference; 95%CI=95% confidence interval].
Characteristics | CTS symptoms | CTS confirmed by NCS | ||||||||||||
---|---|---|---|---|---|---|---|---|---|---|---|---|---|---|
|
|
|||||||||||||
Univariate analysis | Multivariate modela | Univariate analysis | Multivariate modela | |||||||||||
|
|
|
|
|||||||||||
Cases (N=431) | HR | 95% CI | P for trend | HR | 95% CI | P for trend | Cases (N=126) | HR | 95% CI | P for trend | HR | 95% CI | P for trend | |
Sex | ||||||||||||||
Male | 116 | 1.00 | Ref. | 1.00 | Ref. | 31 | 1.00 | Ref. | 1.00 | Ref. | ||||
Female | 315 | 2.23 | 1.31–3.80 | 1.98 | 1.53–2.56 | 95 | 2.15 | 1.65–2.80 | 1.91 | 1.26–2.90 | ||||
Age (years) | <0.001 | <0.001 | <0.001 | <0.001 | ||||||||||
Up to 35 | 117 | 1.00 | Ref. | 1.00 | Ref. | 20 | 1.00 | Ref. | 1.00 | Ref. | ||||
36–45 | 170 | 1.76 | 1.46–2.12 | 1.65 | 1.42–1.92 | 51 | 2.47 | 1.62–3.77 | 2.20 | 1.32–3.67 | ||||
46–55 | 128 | 2.26 | 1.67–3.07 | 1.89 | 1.62–2.21 | 51 | 4.90 | 2.91–8.25 | 3.85 | 2.10–7.08 | ||||
>55 | 16 | 3.89 | 1.71–8.88 | 3.17 | 1.86–5.40 | 4 | 6.63 | 3.14–14.0 | 4.89 | 2.86–8.37 | ||||
Body mass index (kg/m2) | <0.001 | 0.017 | <0.001 | <0.001 | ||||||||||
<25 | 261 | 1.00 | Ref. | 1.00 | Ref. | 60 | 1.00 | Ref. | 1.00 | Ref. | ||||
25.0–29.9 | 127 | 1.39 | 1.13–1.71 | 1.42 | 1.19–1.70 | 45 | 2.16 | 1.56–2.99 | 2.04 | 1.48–2.81 | ||||
≥30.0 | 43 | 1.60 | 1.04–2.47 | 1.47 | 0.85–2.53 | 21 | 3.63 | 2.05–6.43 | 3.04 | 1.48–6.23 | ||||
Predisposing diseasesb | ||||||||||||||
0 | 378 | 1.00 | Ref. | 1.00 | Ref. | 107 | 1.00 | Ref. | 1.00 | Ref. | ||||
≥1 | 53 | 2.47 | 2.02–3.02 | 1.65 | 1.21–2.24 | 19 | 2.96 | 1.63–5.37 | 1.52 | 0.82–2.83 | ||||
ACGIH TLV® categories | <0.001 | <0.001 | 0.005 | 0.001 | ||||||||||
Below the AL | 187 | 1.00 | Ref. | 1.00 | Ref. | 51 | 1.00 | Ref. | 1.00 | Ref. | ||||
Between AL and TLV | 107 | 2.37 | 1.59–3.54 | 2.18 | 1.86–2.56 | 36 | 2.24 | 1.22–4.10 | 1.93 | 1.38–2.71 | ||||
Above TLV | 137 | 2.11 | 1.35–3.28 | 2.07 | 1.52–2.81 | 39 | 2.02 | 1.17–3.49 | 1.95 | 1.27–3.00 |
Based on the reported multivariate risk estimates (table 2), we calculated the fractions of cases attributable in our study population to each risk factor (table 3). For both case definitions, about one third of the cases could be attributed to biomechanical exposure levels above the AL or female gender. Age >35 years contributed to more than 50% of CTS confirmed by NCS and one third of CTS symptoms. BMI ≥25 kg/m2 determined one third of CTS confirmed by NCS, but was implied only in the genesis of the 12% of CTS symptoms. The presence of predisposing pathologies was responsible for a limited proportion of cases. We further estimated for each case the relative contribution of each risk factor to the occurrence of the disease (figure 2). Biomechanical exposure above the AL established by the ACGIH TLV® method was the most important contributing factor (54% of cases) when investigating CTS symptoms; however, the proportional contribution decreased dramatically when investigating CTS confirmed by NCS and this exposure was the main contributing factor only in 6% of subjects affected by CTS (data not shown). Indeed, CTS confirmed by NCS was mainly related to age >35 years (main contributor in the 78.6% of the cases) and BMI ≥25 kg/m2 (11.9% of the cases) (data not shown).
Table 3
Population attributable fractions (PAF) of CTS. Maximum likelihood estimates from the multivariate models presented in table 2. [ACGIH=American Conference of Government Industrial Hygienists; AL=action limit; CTS=carpal tunnel syndrome; NCS=nerve conduction studies;TLV=threshold limit value; 95%CI=95% confidence interval].
Characteristics | CTS symptoms | CTS confirmed by NCS | ||
---|---|---|---|---|
|
|
|||
PAF % | 95% CI | PAF % | 95% CI | |
Female gender | 34.5 | 21.8–45.1 | 33.7 | 11.8–50.1 |
Age >35 years | 31.1 | 23.1–38.3 | 53.7 | 29.6–69.5 |
Body mass index of ≥25 kg/m2 | 12.0 | 0.0–18.6 | 29.9 | 15.6–41.7 |
Presence of predisposing diseasesa | 4.4 | 1.4–7.4 | 4.8 | -3.9–12.7 |
ACGIH TLV® method, exposure above the AL | 30.6 | 21.8–38.5 | 28.2 | 16.3–38.4 |
Figure 2
Proportional contribution to subjects affected by CTS symptoms and subjects affected by CTS confirmed by NCS for selected risk factors. Estimates derived from the multivariate hazard ratios reported in table 2. [CTS=carpal tunnel syndrome; NCS=nerve conduction studies]
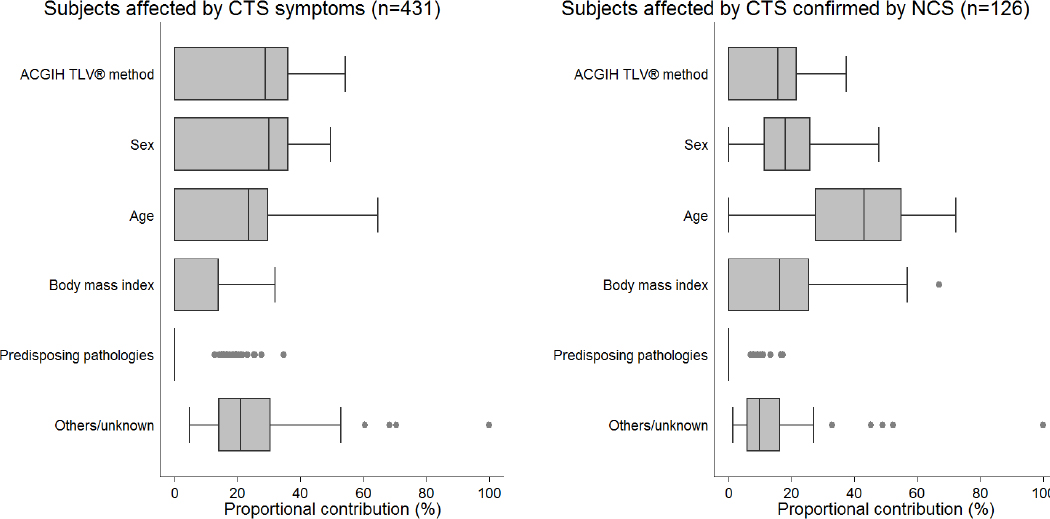
Concerning the study of biomechanical exposure indexes, table 4 presents the estimates from proportional hazards regression models where nPF and HAL were introduced separately or jointly. Whereas the HR for HAL were comparable for both outcomes, nPF was clearly associated only with the occurrence of CTS confirmed by NCS. Of note, we tested for multiplicative interaction between HAL and nPF, but the product terms did not reach the statistical significance threshold (P<0.05) for the inclusion in the final regression models (P-value of the interaction term in multivariable models: CTS symptoms, 0.513; CTS confirmed by NCS, 0.428, data not shown).
Table 4
Estimates from eight proportional hazards regression models adopting different metrics for occupational exposure to biomechanical risk factors. The OCTOPUS cohort, Italy, 2000-2011. [ACGIH=American Conference of Industrial Hygienists; AL=action limit; HAL=hand activity level; HR=hazard ratio; NCS=nerve conduction studies; nPF=normalized peak force; Pyrs=person-years; Ref=reference category; TLV=threshold limit value; 95% CI=95% confidence interval].
Exposure metric | CTS symptoms | CTS confirmed by NCS | ||||||||||||
---|---|---|---|---|---|---|---|---|---|---|---|---|---|---|
|
|
|||||||||||||
Crude estimates | Adjusted estimates | Crude estimates | Adjusted estimates | |||||||||||
|
|
|
|
|||||||||||
Cases (N=431) | HR | 95%CI | P for trend | HRa | 95%CIa | P for trend | Cases (N=126) | HR | 95%CI | P for trend | HRa | 95%CIa | P for trend | |
MODEL A, ACGIH TLV® categories | <0.001 | <0.001 | 0.005 | 0.001 | ||||||||||
Below AL | 187 | 1.00 | Ref. | 1.00 | Ref. | 51 | 1.00 | Ref. | 1.00 | Ref. | ||||
Between AL and TLV | 107 | 2.37 | 1.59–3.54 | 2.18 | 1.88–2.56 | 36 | 2.24 | 1.22–4.10 | 1.93 | 1.38–2.71 | ||||
Above TLV | 137 | 2.11 | 1.35–3.28 | 2.07 | 1.52–2.81 | 39 | 2.02 | 1.17–3.49 | 1.95 | 1.27–3.00 | ||||
MODEL B (HAL) | <0.001 | <0.001 | 0.006 | <0.001 | ||||||||||
1.0–3.0 | 170 | 1.00 | Ref. | 1.00 | Ref. | 44 | 1.00 | Ref. | 1.00 | Ref. | ||||
3.1–5.0 | 213 | 2.03 | 1.38–3.01 | 2.10 | 1.61–2.73 | 60 | 2.17 | 1.43–3.31 | 2.06 | 1.61–2.65 | ||||
5.1–8.5 | 48 | 2.41 | 1.50–3.87 | 2.05 | 1.54–2.74 | 22 | 2.38 | 1.15–4.95 | 2.06 | 1.37–3.09 | ||||
MODEL C (nPF) | 0.004 | 0.014 | 0.001 | <0.001 | ||||||||||
1.0–3.0 | 69 | 1.00 | Ref. | 1.00 | Ref. | 18 | 1.00 | Ref. | 1.00 | Ref. | ||||
3.1–5.0 | 293 | 1.55 | 1.28–1.89 | 1.24 | 0.93–1.66 | 86 | 1.93 | 1.19–3.12 | 1.68 | 0.87–3.23 | ||||
5.1–7.0 | 69 | 1.62 | 1.13–2.32 | 1.54 | 1.10–2.16 | 22 | 2.64 | 1.54–4.51 | 2.62 | 1.63–4.21 | ||||
MODEL D | <0.001 | <0.001 | 0.071 | 0.030 | ||||||||||
HAL | ||||||||||||||
1.0–3.0 | 170 | 1.00 | Ref. | 1.00 | Ref. | 44 | 1.00 | Ref. | 1.00 | Ref. | ||||
3.1–5.0 | 213 | 2.29 | 1.54–3.39 | 2.24 | 1.80–2.79 | 60 | 2.15 | 1.40–3.31 | 1.97 | 1.63–2.38 | ||||
5.1–8.5 | 48 | 2.72 | 1.56–4.74 | 2.31 | 1.80–2.96 | 22 | 2.18 | 0.91–5.25 | 1.79 | 1.06–3.03 | ||||
nPF | 0.980 | 0.625 | 0.048 | 0.007 | ||||||||||
1.0–3.0 | 69 | 1.00 | Ref. | 1.00 | Ref. | 18 | 1.00 | Ref. | 1.00 | Ref. | ||||
3.1–5.0 | 293 | 1.50 | 1.26–1.78 | 1.19 | 0.98–1.44 | 86 | 1.76 | 1.09–2.86 | 1.60 | 0.94–2.71 | ||||
5.1–7.0 | 69 | 0.93 | 0.56–1.52 | 0.89 | 0.58–1.38 | 22 | 1.53 | 0.85–2.77 | 1.70 | 1.08–2.69 |
Discussion
With this report on the complete follow-up of the OCTOPUS cohort, we were able to confirm the main findings of our previous accounts, ie, that the ACGIH TLV® metrics correctly predicts that, when the TLV is exceeded, a sizable increase (roughly two-fold) in the risk of CTS can be expected. Our result is stable and robust, being based on a follow-up extended up to ten years, 8883 total person-years of observation and 126 cases defined according to a state-of-the art clinical definition of the outcome based on NCS (8000 person/years and 431 cases if considering the case definition based on symptoms only).
Past investigations on CTS suggested that more that 50% of the cases might be attributable to occupational factors (28). However, in this highly informative study conducted among industrial and service workers we found that no more than one third of the cases could be attributed to exposure levels above the ACGIH AL. Moreover, biomechanical risk factors captured by the ACGIH TLV® method seldom were the most important contributing factors to CTS cases (although in many patients it appeared to be the most important one for the onset of CTS symptoms with negative NCS). On the one hand, our findings suggest that preventive efforts targeted at reducing workload on upper limbs may substantially reduce the burden of CTS. On the other hand, the major role of personal risk factors in the genesis of CTS hampers the retrospective assessment of the occupational origin of this disease; indeed, the proportional contribution – that represents a lower bound estimate for the probability of causation (27) – is often well below 50% also in a population mainly composed by blue-collar workers.
Compared to our previous report on the first two years of observation of the cohort (19), this extended follow-up documented a previously non-observed result, that is a small reduction in the incidence of CTS with the highest level of exposure (above the TLV) when compared to the intermediate level of exposure (between the AL and the TLV). Whereas this finding is new for our cohort, it has already been reported in the US study on CTS (20). Of note, the relative magnitude of the risk estimates in both our study and the US one is comparable, giving additional solidity to both results. These observations might be explained by the well-known “healthy worker survivor effect”, that is the tendency for subjects more susceptible to a certain disease to exit a cohort, especially if the observation period is long enough compared to the natural history of the disease (29, 30). That may well be the case with CTS, a disease in which the relevant period of exposure may be relatively short (as demonstrated by the pregnancy-associated CTS) (31). Of note, in a supplementary analysis of the incidence of CTS symptoms in our cohort between 2003 and 2011, we found an incidence rate (IR) of 4.7 per 100 person-years among workers who decreased their exposure level (ie, ACGIH TLV® category) over the study period and an IR of 2.7 per 100 person-years among those who kept a stable level of exposure (data not shown). This observation seems to support the hypothesis of a healthy worker survivor effect. Were this the case, we might have slightly underestimated the incidence of CTS. Indeed, some workers might have quit the job during the study period due to CTS; in our analysis, these subjects would be included among the lost-to-follow-up. Of note, our cohort included many workers with relatively long job tenures (≥7 years). This fact increases the possibility that one of the components of the healthy worker effect – namely the continuing employment of healthy individuals – might affect our estimates (32). Of course, other alternative explanations still held validity, such as a possible misclassification of exposure, or an issue with the predictive power of the ACGIH TLV® method (but this would not explain why in the previous report, based on the same exposure data, the effect was not observed), and, of course, a chance effect should not even be overlooked: all considered, however, based on our present knowledge, a “healthy worker survivor effect” seems to be a likely explanation for the pattern observed.
Another finding which needs to be mentioned is that, differently from the US study, nPF did not seem to correlate with the incidence of CTS better than HAL (20). However, by definition, HAL is computed taking into consideration “exertion frequency and duty cycle (% of work cycle where force is >5% of maximum)”, whereas nPF is irrespective of the duration of the exertion in relation to that of the work cycle (22). So, it may be that in work cycles characterized by a relatively elevated HAL, but where the duration of the most forceful exertion (nPF) is relatively short, HAL may capture the total “load” received by the hand better than nPF. Another issue to be considered is that the distribution of exposure in our study seems to be more skewed towards groups with elevated HAL and relatively lower PF than the US study, including fewer subjects with relatively high PF and low HAL. Everything else considered, our study confirms that hand activity, performed with some amount of forceful exertions, significantly increases the risk of the disease. Risk assessment methods based on the frequency of “any exertion” might overestimate the risk in work cycles where movements are frequent but the force used is slight.
A recent overview of systematic reviews and a meta-analysis of current research concluded that there is high evidence for an increased risk of CTS in activities requiring a high degree of repetition and forceful exertion while the evidence for vibration and non-neutral wrist postures appears moderate and low respectively (33).
This study and the pooled US analysis (20) provide independent validations of the ACGIH TLV® method by means of the only effective method, that is, a longitudinal cohort study. Some prospective evidence is also available for the Strain Index method (34). Whereas all the other methods to assess workload on upper limbs have been tested, at best, only in cross-sectional studies, which do not provide a suitable validation strategy because they are unable to assess causality (35). On balance, the ACGIH TLV® method for manual work may be considered the one for which the largest body of evidence of predictive value is available, although it seems to need some minor adjustments. Indeed, the excess risk of CTS in workers exposed just above the ACGIH AL, confirmed by both our and US study, calls for a fine-tuning of the TLV, which might not be protective enough for the most susceptible workers (those who may develop the disease at the lower levels of exposure, mostly female workers older than 40 years of age) (20).
Lastly, it should be noted that the incidence of CTS confirmed by NCS reported in our study was considerably higher than in population studies, as the one conducted in Tuscany (3). This can be related to two factors. Firstly, we were actively seeking cases, asking for symptomatic subjects to undergo a NCS, whereas patients who did not seek medical care were not included in the passively collected rates based on cases seen by neurologists in nerve conduction laboratories. Secondly, we did not study the general population, but a population of manual workers, where the CTS incidence is >4 times higher compared to non-manual workers (5).
Study strengths and limitations
Our study is one of the very few prospective validations of observational methods for the assessment of biomechanical exposures at work (35). To our knowledge, the OCTOPUS cohort is larger than any other previously published occupational cohort designed to study the association between CTS and biomechanical risk factors and as large as the pooled US study (20). The exposure assessment was performed using a standardized and fast observational method (22). We analyzed a sample of representative workers to estimate the HAL and nPF associated with each task. A drawback of our approach is the potential for exposure misclassification because we did not account directly for personal and anthropometric characteristics that may influence the intensity of biomechanical exposures (eg, postural changes). Also, on the opposite of the US study, we did not apply objective methods to measure nPF (20). However, while our exposure assessment method does not ensure the absence of exposure misclassification, it does represent a real world setting. In fact, the observational ACGIH TLV® method can be applied on a large scale because it is fast, inexpensive and easily implemented. This fact increases the external validity of our study; our risk stratification based on ACGIH categories could be replicated in many industrial settings.
Another strength of our study is that information on possible confounders was collected prospectively by direct interviews. Regarding participation in the study, on the one hand, the initial response proportion was satisfactory (>94%), on the other hand, loss to follow-up was not negligible and attrition bias could represent a concern. A comparison of baseline characteristics in the OCTOPUS cohort highlighted that women tended to be lost to follow-up more than men. However, we should consider that loss of subjects to follow-up could have been mainly determined by workers who changed their employment status, were from factories that closed down (the underwear factory), or were temporarily absent from work (eg, due to illness, parental leave, or other employee benefits). All the aforementioned conditions seem to be weakly related to CTS: hence, we hypothesize that loss to follow-up should not substantially bias our results. Another possible limitation of our study is that the assessment of biomechanical risk factors was performed only at baseline and at the end of the study, or when we were notified of significant changes in the workplaces followed for the entire period of the study. However, there were no changes in production technologies or volumes in the seven participating enterprises during the study period. Therefore, misclassification of biomechanical exposure due to changes in jobs should be nearly negligible, although we cannot completely rule out the presence of residual confounding due to exposure misclassification for unknown causes. Since exposure assessment was not feasible for multi-task jobs, only activities that included regular or predictable patterns of exertions over the course of each work shift were assessed. Thus, our study is not able to provide information for multi-task manual jobs (eg, maintenance workers, cleaners, janitors). In the OCTOPUS cohort, HAV were registered as a dichotomous exposure; no measurements of frequency or acceleration were collected. Hence, we were not able to study HAV properly as a risk factor for CTS and we only explored the exposure to HAV as a possible confounder of the relationship between the ACGIH classification and the risk of CTS.
We estimated the attributable fractions of CTS. When interpreting these figures, it should be kept in mind that the values for ACGIH TLV® categories might be underestimated due to exposure misclassification – that is more likely to attenuate rather than inflate our estimates. Also, we did not measure some personal (eg, cardiovascular risk factors) and occupational (eg, psychosocial risk factors) characteristics that were recently hypothesized as risk factors for CTS (36, 37). On the one hand, the absence of information on these variables could substantially affect the calculation of attributable fractions only under the unlikely hypothesis that these factors were strong confounders of the association between CTS risk and biomechanical exposures. On the other hand, the proportional contributions determined by each risk factor might be overestimated. Indeed, the proportional contributions are rescaled so that their total always sums up to 100%. Hence, the inclusion of any further risk factors in the multivariable models determines a decrease of the proportional contributions estimated for the other covariates.
Concluding remarks
Based on the measure of the HAL and nPF, the ACGIH TLV® method enables the classification of workers in three categories (below the AL, between the AL and the TLV, above the TLV). The HAL is based on the frequency of hand exertions and the duty cycle (distribution of work and recovery periods), while the nPF is the peak effort exerted by the hand during each regular work cycle. According to the ACGIH, the TLV should not be exceeded; also, the AL is considered the level that triggers general controls, including surveillance. As expected, we found an increased incidence of CTS for workers exposed above the TLV. However, we also observed a non-negligible risk for workers exposed between the AL and the TLV; hence, the current limits – AL and TLV – might not be sufficiently protective for some workers. The growing body of evidence suggests a fine-tuning of the proposed thresholds.