The present study sought to determine whether shift workers exhibit altered pain perception after working night shifts. Prospective studies have found that adverse work schedules, including night-shift work (NSW), increase the risk of musculoskeletal pain after several months (1, 2). Shift workers often report lack of restorative sleep, reduced sleep duration, and poor sleep quality (3–8). Prospective studies indicate that sleep problems are associated with an increased risk for long-lasting musculoskeletal pain (9–15) and experimental studies suggest that even a relatively modest restriction or disturbance of sleep increases pain sensitivity. This is manifested as larger responses to experimentally induced pain (16–21), as increased spontaneous pain (22–24), or as altered pain modulation (23, 25–29). A recent meta-analysis confirm that sleep restriction increases sensitivity for several types of pain (30). Altered pain modulation may be a potential pathogenic mechanism of musculoskeletal pain disorders among shift workers. Hence, there is a need for knowledge of effects of night shifts on pain system function in the short term.
Experimental studies are typically performed on healthy volunteers, presumably not used to being awake at night. It is possible that shift workers will adapt or habituate to sleep restriction, becoming more robust to the hyperalgesic effect of sleep restriction. Furthermore, working night shifts may affect circadian rhythms differently than experimental sleep restriction. To the authors’ knowledge, there is no experimental study of effects of night-work-induced sleep restriction on pain sensitivity or pain modulation.
Thus, the aim of the present study was to determine whether NSW alters pain perception and pain modulation.
Methods
Subjects
Nurses were recruited by wall postings or brief bulletins at the hospitals’ intranet pages at major hospitals in the Oslo area. Fifty-eight self-reported healthy nurses volunteered for the experiment (figure 1). Five subjects withdrew before the first experimental day, 53 subjects participated in the first sleep condition [mean 31.6, standard deviation (SD) 9.0 years; range 24–57; 41 women] and 40 subjects participated in both sleep conditions. Of the 13 subjects participating in only one sleep condition, 8 dropped out after the habitual sleep condition and 5 dropped out after the night shift condition. Despite an unbalanced dataset, data from all 53 subjects was analyzed since complete case analysis is generally assumed to reduce the robustness of the estimates (31). Due to technical difficulties in some of the procedures, the number of subjects was not the same across outcome measures. A priori power analysis based on a previous study from our laboratory (32) showed that 25 subjects were needed to detect a difference of 1 cm in pain inhibition on a 10 cm visual analog scale (VAS) between the two protocols with a standard deviation of 1.5, assuming a two-sided significance level of 5% and a power of 90%.
Figure 1
Flowchart of study participants. Five subjects withdrew before the pre-test.a Habitual sleep participants (N=27), night shift participants (N=25).b Reasons for dropout after the first sleep condition: personal (N=11), pregnancy (N=2).c Habitual sleep participants (N=21), night-shift work participants (N=19).
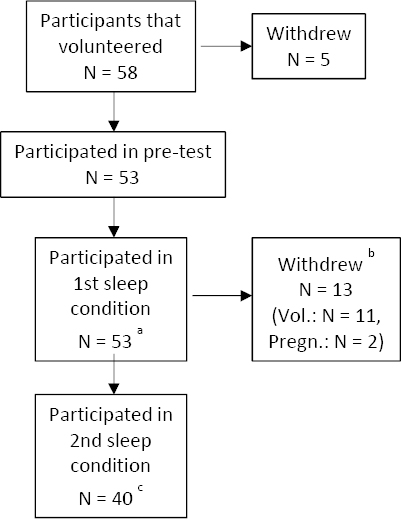
Of the 53 subjects, 39 followed a rotating shift schedule (morning, evening, night), 6 were permanent night-workers and 8 had an unknown shift schedule. Exclusion criteria were: pain with intensity ≥3/10 lasting ≥3 months during the last two years, having psychiatric, neurologic, heart or lung disease (well-regulated asthma allowed), headache of moderate intensity for >2 days per month on average, regular use of over-the-counter analgesics, hypertension (>140/90 mmHg), being pregnant or breast feeding.
All participants received written information and signed an informed consent form. The Norwegian Regional Committee for Medical Research Ethics approved the study (Region South-East B, approval # 2012/199).
Design
The study was a paired cross-over design with block randomization and consisted of two experimental sessions with different sleep condition: after at least two nights with habitual sleep and after two consecutive nights at work, each subject working at his/her regular work place. Except for three subjects having their last night shift three days before the habitual sleep condition, all subjects had ≥4 nights with habitual sleep before the experiment. Most of the subjects (N=29) worked two consecutive nights before the NSW experiment, 13 subjects worked three consecutive nights and 3 subjects worked four consecutive nights. Subjects were instructed to abstain from alcohol the 24 hours prior to the lab experiment. For the NSW condition, the subjects came directly from work to the experiment. All work sites were located <25 km from the research institute, meaning ≤60 minutes with public transport.
Procedure
Subjects were familiarized with the experimental procedure on a pre-test session, two days before the first experimental session. The second experimental session occurred mean 38.1 (SD 39.6) days after the first. The two experimental sessions were identical except for the sleep condition. The same female experimenter tested all participants and was blinded with respect to the sleep condition, giving instructions from a written protocol. The adequacy of the blinding procedure was confirmed by testing on a subset of subjects. The experiment started between 08:00 and 09:00 hours. After ≥5 minutes rest in a seated position, blood pressure was measured three times (Dinamap V100, GE Healthcare) and averaged. Subjective and objective measures of sleepiness were obtained before the experiment. The experimental pain stimuli were delivered in this sequence: pressure pain, 60 electrical pain stimuli, heat pain and finally heat pain in parallel with cold pain. For more details on the experimental pain protocols, see (18, 32).
Sleep, sleepiness and vigilance measurements
At inclusion, habitual daytime sleepiness was measured with the Epworth Sleepiness Scale (ESS) (33) and a composite measure of sleep disturbance was obtained with the Pittsburg Sleep Quality Index (PSQI) (34). Sleep was monitored via a sleep diary and an accelerometer device worn on the non-dominant ankle (ActiSleep, Actigraph LLC, Pensacola, Florida, USA) 24 hours prior to each experiment. The sleep diary was smartphone-based (paper-based for eight subjects not owning a smartphone). Subjects entered bedtime (lights off), rise time (lights on), and naps in the sleep diary. Actigraphy-based sleep analysis was performed by the Cole-Kripke algorithm (Actilife software v. 6.12.0, Actigraph LLC, Pensacola, Florida, USA). Actigraph recordings were obtained before both experimental sessions in 36 subjects, before one of the sleep conditions in 15 subjects, and, due to technical difficulties, not at all in 2 subjects. In each experimental session, sleepiness was measured subjectively by the Karolinska sleepiness scale (KSS) (35) and vigilance was measured by a computerized version of the 10-minute psychomotor vigilance test (PVT) (custom-written C++ program, National Institute of Occupational Health, Oslo, Norway). We used the mean inverse reaction time from the PVT as a parameter of vigilance, which has been shown to be particularly sensitive to sleep restriction (36).
Experimental pain stimuli
The subjects participated in standardized tests of electrical pinprick pain, contact heat pain, cold pain, and pressure pain. In addition, pain inhibition was tested by the conditioned pain modulation (CPM) paradigm (37).
Electrical stimulation
High-density electrical stimulation was delivered by a constant current stimulator (DS7A and DG2A, Digitimer, Hertfordshire, England) through a platinum pin electrode (cathode, length: 0.2 mm Ø: 0.2 mm) attached to the skin by double-adhesive tape at the volar forearm 10 mm medial to half the distance between the insertion point of the biceps brachii tendon and the distal end of ulna (32). The anode was a conductive Velcro-strap (Alpine Biomed ApS, Skovlunde, Denmark) soaked in an isotonic NaCl solution at the ipsilateral upper arm. Each stimulus consisted of two unipolar 0.5 ms pulses at an interval of 10 ms. Individual pain threshold was determined by a sequence of three ascending series of stimuli, increased by steps of 0.1 mA (from 0 mA). The pain threshold was calculated as the mean of the two last thresholds. Sixty stimuli were presented, equally divided between three intensities, 2 × pain threshold (A), 3 × pain threshold (B) and 4 × pain threshold (C). Pain intensity was rated on a computerized 10 cm visual analogue scale (VAS) with endpoints “not painful” and “worst imaginable pain”.
Heat stimulation
Heat pain was delivered by a 12.5 cm2 peltier thermode (MSA-II, Somedic AB, Solna, Sweden) attached to the volar forearm by a blood pressure cuff (20 mmHg). The stimulus temperature was individualized, defined as the temperature that induced a pain intensity of 6 (“pain6”) on a 0–10 verbal numerical rating scale (NRS, endpoints 0=“no pain”, 10=“worst imaginable pain”). The pain6 temperature was determined in a pre-test session and the same temperature was used for both experimental sessions. Heat pain was rated continuously (VAS, 10 cm, end points “no pain” and “worst imaginable pain”).
Cold stimulation
Cold stimulation was performed by immersion of the hand up to the wrist in 7 °C circulating water (Lauda Alpha RA8, lauda-brinkmann.com), with fingers spread for 2 minutes, and verbal pain scores were obtained at 30-second intervals (0–10 NRS).
Pain inhibition
In the conditioned pain modulation paradigm, the change in pain response to a painful test stimulus (TS) induced by another painful conditioning stimulus (CS) is an indicator of endogenous pain inhibition (32, 37, 38). The 2-minute heat stimulation served as TS and the contralateral 2-minute cold stimulation served as CS. TS was first presented alone. After a 5-minute break, TS + CS was presented simultaneously.
Pressure pain stimulation
Pressure was induced by a handheld pressure algometer (Wagner Force One model FDIX, Wagner Instruments, Greenwich, CT, USA; probe size 1 cm2). A pilot study indicated that pressure pain threshold repeatability was better for the trapezius muscle compared to other muscles tested. The test site was found at 1/3 the distance along an imaginary line connecting the C7 spinous process and acromion. The algometer output was sampled by a computer (custom-written C++ program, National Institute of Occupational Health, Oslo, Norway). The pressure started at 0 kPa and continued until the subject reached a score of 5 cm on a 0–10 cm VAS with end points “no pain” and “worst imaginable pain”. Visual feedback on the applied pressure was used to ensure increasing pressure at a steady rate (aimed at 50 kPa/sec). The procedure was repeated three times. The response to two pressure pain intensities was assessed: the pressure pain threshold (PPT) and the pressure equal to a VAS rating of 5 cm (PP5) on a 0–10 cm VAS. The latter was based on a linear fit between pressure and pain.
Data analysis
Total sleep time, time in bed, number of awakenings, and sleep efficiency were calculated based on the actigraph measurements. Times for lights-off and -on from the last 24 hours were entered manually offline based on the sleep diary. Only main sleeps periods were analyzed by the Cole-Kripke algorithm, whereas daytime napping (seven subjects) was noted in the sleep diary and added to the total sleep time for these subjects.
Electrical pain scores were averaged for each of the three stimulus intensities. Heat pain scores (stored at 1-second intervals) were processed by averaging scores for every 5 seconds, excluding the first 20-second ramp period. This left 20 VAS scores across the 2-minute stimulus period for each subject and condition. The pain inhibitory effect was defined as the difference between the mean VAS rating over the 2-minute TS and TS+CS conditions (VASTS + CS – VASTS). A negative value corresponded to an inhibitory modulatory effect of CS on TS pain ratings. For cold pain, all four pain scores were entered into the statistical model. Similarly, for pressure pain, all three repetitions were entered into the statistical model.
Statistical analysis
Based on visual inspection of the histograms, most of the sleep variables were non-normally distributed. Thus, the paired comparison between sleep conditions was performed with the non-parametric Wilcoxon test for these variables.
Sleep condition (habitual sleep versus NSW) was the primary fixed factor for each pain outcome (electrical pain score, heat pain score, cold pain score, PPT, PP5 and pain inhibition). Given the repeated measures nature of the data, time was added as a fixed factor for heat pain (20 measurements), cold pain (four measurements), PPT and PP5 (three measurements). The pain inhibitory effect was tested by adding the heat pain score during cold pain to the model as a second fixed factor (inhibition), including the sleep × inhibition interaction. All analyses were performed with linear mixed models with an unstructured covariance structure and following the same general procedure. Firstly, which of these factors to include as random slope were determined: sleep condition, stimulus intensity (electrical pain only), and time (heat pain, cold pain, pressure pain). Secondly, sleep condition was entered as a fixed factor. For electrical pain, stimulus intensity was an additional fixed factor. Random intercept for subject was included in all models. The decision of which of the fixed and random factors to keep in each model was based on the Akaike Information Criterion. Maximum likelihood estimation was used for initial test, and restricted maximum likelihood estimation was used for the final models. The fit of each model was tested by visual observation the Q-Q plot of the residuals as an indicator of normality. All statistical analyses were performed in Stata v.13 (StataCorp, College Station, TX, USA).
Results
Sleep disturbance, daytime sleepiness and blood pressure
Habitual Pittsburgh Sleep Quality Index (PSQI) scores were available from 24 subjects and ranged from 1–10 (mean 4.9, SD 2.7). Twelve subjects had a PSQI score of ≥5, indicating poor sleep quality. ESS were available from 39 subjects and ranged from 1–16 (mean 7.3, SD 3.6). Seven subjects had a score of ≥11 indicating high daytime sleepiness. Subjects were normotensive (mean systolic blood pressure 114.8 (SD 9.6) mmHg and mean diastolic blood pressure 68.7 (SD 7.7) mmHg).
Sleepiness, reaction time and sleep/wake parameters
Subjective sleepiness increased by 2.8 points (1–9 scale), whereas reaction time increased by 0.03 seconds after NSW versus after habitual sleep (table 1). During the last sleeping period before each experimental session, subjects in the NSW condition slept one hour shorter and had approximately 1.5 fewer awakenings versus the habitual sleep condition, whereas sleep efficiency did not differ between sleep conditions (table 1). Median, minimum, and maximum times for lights-off and -on, as well as time awake before the experiment, are reported in table 1.
Table 1
Descriptive statistics of subjective sleepiness, reaction time, actigraph-based sleep measures, lights-off, lights-on and time awake. Descriptives are made on the full sample, whereas z and P values are from the sample for which both conditions are avaliable. [KSS= Karolinska Sleepiness Scale (1–9); SD=standard deviation]
Habitual sleep (HS) | Night-shift work (NSW) | za | P-valuea | |||||||
---|---|---|---|---|---|---|---|---|---|---|
|
|
|||||||||
Mean | SD | Median | Min–Max | Mean | SD | Median | Min–Max | |||
Subjective sleepiness (KSS) | 4.10 | 1.73 | 6.87 | 1.04 | -5.3 | <0.001 | ||||
Reaction time (seconds)b | 0.38 | 2.34 | 0.41 | 1.80 | 3.1 | 0.002 | ||||
Time-in-bed (hours)c | 7.7 | 1.2 | 6.6 | 1.6 | 3.4 | <0.001 | ||||
Total sleep time (hours)d | 7.5 | 1.3 | 6.5 | 1.5 | 3.1 | 0.002 | ||||
Number of awakeningsd | 6.1 | 4.9 | 4.6 | 3.6 | 2.7 | 0.007 | ||||
Sleep efficiency (%)d | 96.4 | 2.8 | 95.8 | 3.2 | -0.3 | 0.730 | ||||
Lights-off time (hours) | 22.7 | 22.2–3.7 | 9.0 | 4.0– 11.0 | ||||||
Lights-on time (hours) | 7.5 | 4.8– 12.0 | 15.5 | 12.0– 19.0 | ||||||
Time awake (hours)e | 1.4 | 0.6– 3.8 | 17.0 | 13.3– 21.0 |
Pain intensity
Pain after electrical stimulation was scored 22.3 % higher in the NSW condition relative to the habitual sleep condition (table 2). The estimated effect size was 0.51 cm (95% CI 0.24–0.77) on the 0–10 cm VAS (table 3). Pain scores also increased with increasing stimulus intensity. The estimated effect size was 0.91 cm (95% CI 0.70–1.12) on the 0–10 cm VAS for an increase in one stimulus intensity unit (table 3). There was no interaction between sleep condition and stimulus intensity (P>0.25).
Table 2
Descriptive statistics showing pain scores in response to experimental pain stimuli by sleep condition. [SD=standard deviation; VAS=visual analog scale; NRS=numerical rating scale]
Habitual sleep (HS) | Night-shift work (NSW) | Delta valuea | Percent changeb | |||||
---|---|---|---|---|---|---|---|---|
|
|
|||||||
N | Mean | SD | N | Mean | SD | |||
Electrical pinprick pain, VASc | 40 | 2.20 | 1.76 | 37 | 2.69 | 1.74 | 0.49 | 22.3 |
Heat pain (VAS)c | 46 | 4.68 | 2.84 | 41 | 5.92 | 2.80 | 1.24 | 26.5 |
Cold pain (NRS)d | 48 | 6.75 | 2.17 | 45 | 7.04 | 2.13 | 0.29 | 4.3 |
Pressure pain threshold (Newton) | 46 | 30.8 | 14.8 | 45 | 30.3 | 13.3 | -0.59 | -1.9 |
Pressure pain at VAS 5/10 (Newton) | 46 | 82.4 | 60.4 | 44 | 79.4 | 48.2 | -3.06 | -3.7 |
Pain inhibition (VAS)c | 46 | -1.12 | 1.85 | 41 | -1.88 | 1.79 | -0.75 | 66.9 |
Table 3
Statistical summary after linear mixed models analyses by experimental pain type. Random intercept for subject was included in all models. Random slope for time was included for heat pain, cold pain, pressure pain and pain modulation. Random slope for sleep was included for electrical pain and cold pain. The coefficient (estimated effect size) is in unit cm for electrical pain, heat pain, cold pain and pain inhibition, and in Newton for the pressure pain measures. [Obs=number of observations per sleep condition. 95% CI=95% confidence interval; VAS=visual analog scale].
Pain type and fixed factor | Obs | Coefficient | 95 % CI | P-value |
---|---|---|---|---|
Electrical pinprick pain | 231 | |||
Sleep condition | 0.51 | 0.24–0.77 | <0.001 | |
Stimulus intensitya | 0.91 | 0.70–1.12 | <0.001 | |
Heat pain | 1704 | |||
Sleep condition | 1.24 | 0.52–1.96 | 0.001 | |
Cold pain | 357 | |||
Sleep condition | 0.13 | -0.56–0.30 | 0.566 | |
Pressure pain threshold | 269 | |||
Sleep condition | -0.10 | -1.82–2.03 | 0.917 | |
Pressure pain at VASb 5/10 | 264 | |||
Sleep condition | -0.24 | -5.66–5.19 | 0.932 | |
Pain inhibition | 3444 | |||
Sleep condition | 0.85 | 0.28–1.42 | 0.003 | |
Inhibition | -1.13 | -1.53– -0.74 | <0.001 | |
Sleep condition × inhibition | -0.71 | -0.85– -0.57 | <0.001 |
Heat pain was scored 26.5% higher in the NSW condition relative to the habitual sleep condition (table 2 and solid symbols in figure 2A). The estimated effect size was 1.24 cm (95% CI 0.52–1.96) on the 0–10 cm VAS (table 3).
Figure 2
(A) Heat pain (TS) by sleep and conditioned pain modulation (CPM) conditions. Data are displayed as the median, interquartile range (box), 10–90% confidence intervals (leaf) and 5–95% confidence intervals (dots). There was a main effect of sleep on TS heat pain alone (#:P=0.001), a main effect of CPM alone, TS+CS pain was rated lower than TS pain alone (*:P<0.001) and a sleep × CPM interaction, the CPM effect being stronger after NSW versus after HS ($:P=0.0001). (B) CPM-effect by sleep condition. [CS=conditioning stimulus (cold); HS=habitual sleep; NSW=night shift work. VAS=visual analogue scale.]
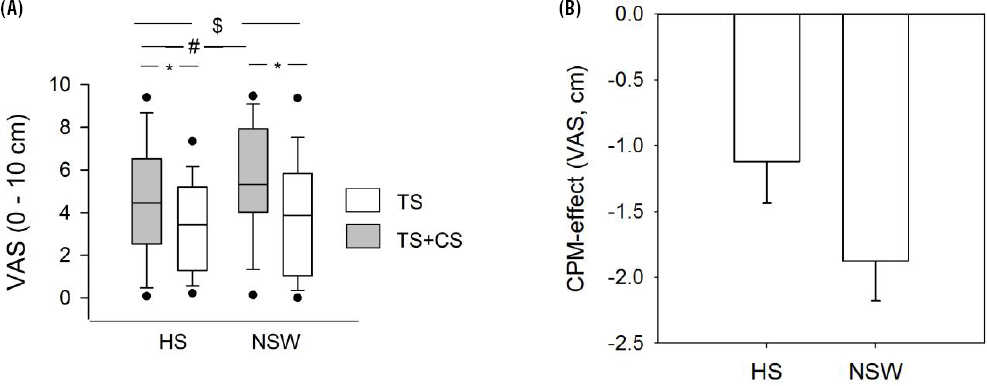
Cold pain sensitivity was scored 4.3% higher in the NSW condition relative to the habitual sleep condition (table 2), corresponding to a non-significant estimated effect size of 0.13 on the 0–10 NRS (table 3).
Pressure pain sensitivity was not significantly associated with sleep condition; the pressure pain threshold decreasing by 1.9 % and pressure pain at VAS 5/10 decreasing 3.7 % (table 2), corresponding to estimated effect sizes of -0.1 N and -0.24 N, respectively (table 3).
Pain modulation
There was a significant pain inhibitory effect of the conditioning cold pain stimulus on heat pain; heat pain was rated lower when given in parallel with cold pain, than when given alone, corresponding to an estimated effect size of -1.13 cm on a 0–10 cm VAS (table 3, open symbols in figure 2A). There was also a significant sleep × CPM interaction, the pain inhibitory effect was -1.12 cm in the habitual sleep condition versus -1.88 cm in the NSW condition (figure 2B), corresponding to an estimated effect size of -0.71 cm on a 0–10 cm VAS (table 3).
Discussion
The present study of shift workers found that two consecutive night shifts increased pain scores to experimental electrical stimulation and heat with clinically relevant effect sizes, whereas experimental cold pain and pressure pain were not significantly changed. The study also showed that NSW increased endogenous pain inhibition.
Effect of night shift work on sleep measures
The present subjective sleepiness of night-shift-induced sleep restriction is comparable to that reported by experimental sleep restriction studies (17–19), indicating that experimental sleep restriction studies may be a valuable model to study the effect of night-shift-induced effects on sleep.
Effect of night-shift work on experimental pain
The paired comparison between the response to experimental pain the morning after two nights at work and after two nights with habitual sleep showed that ratings of electrically induced pain increased by 22.3%. This is larger than findings from a previous study from our lab, where a slightly different protocol yielded an increase of 8% (18).
Elevated heat pain sensitivity after NSW (approximately 24%) is comparable to several studies of experimental sleep restriction, using either contact heat or laser stimulation (17, 19–21, 32, 39–41), although no effect of sleep restriction have been reported on heat-pain tolerance level (42).
An increased pain inhibitory effect following NSW contrasts with several experimental sleep restriction studies (25–27, 29) but is in accordance with a previous study from our lab (32) and with another study that points in the direction of increased pain inhibition after sleep restriction (23). One should keep in mind that endogenous pain modulation is not an unambiguous phenomenon, and that test paradigms vary considerably making comparisons across studies challenging (38, 43).
Cold-pain sensitivity after NSW and sleep restriction has been little investigated, but lowered cold pain thresholds have been reported in at least two previous studies (17, 41). The present study found that supra-threshold pain reports were unaffected by NSW. Of note, the present 2-minute cold-water immersion was given in parallel with heat pain in the CPM protocol. Subjects were told to focus on the heat pain rating, which may have rendered the cold pain ratings less accurate.
Our finding of unchanged pressure-pain sensitivity following NSW contrasts with earlier studies of experimental sleep restriction (18, 19, 42). Relative to the previous study from our lab, the present study population were somewhat older. People tend to be less sensitive to experimental pain with increasing age (44). Another difference is the availability for the shift workers to reduce the sleep debt with recovery sleep between the two nights at work. Circadian factors not accounted for in the present study may potentially also affect pressure pain and cold pain differently than electrical and heat pain.
Methodological considerations
Because the number of waking hours before testing differed between sleep conditions, one may argue that the experiments should have been performed after recovery sleep following the second night shift. However, the long waking time cannot fully explain the results. In experimental studies, partial sleep restriction (of the first part of the night) induces hyperalgesia in the same manner as full sleep deprivation (18). In addition, in the partial sleep restriction studies, the time awake has been the same for both the exposure and the control condition. However, we have not been able to control for other factors related to circadian rhythm disruption and this is a weakness of the study. Little documentation exist on variation in pain sensitivity due to circadian phase, but one study found only small differences in heat and cold pain sensitivity (45). The number of pain tests is relatively high and one cannot exclude possible carry-over effects between tests. However, this is not likely to have affected our main finding as the order of the tests was fixed.
The subjects of the present study were adults, representative of the working population and somewhat older than subjects in many experimental sleep restriction studies. There is a possibility that subjective health complaints that typically increase with age interact with the effects of the intervention to be tested. On the other hand, revealing significant effects in such a group increases external validity. Another strength of the present study is the use of a sleep diary verified by actigraphy.
Concluding remarks
To our knowledge, the present study is the first to show that professional shift workers exhibit an increased sensitivity to pain after NSW. Moreover, we report differential effects on different pain modalities, and explaining this should be a focus for future studies. Lowered pain threshold increase the risk for sensitization of pain pathways. Accordingly, the findings may indicate that healthy workers are at increased risk for development of pain complaints after night shift, and workers with existing pain symptoms may be at risk for exaggeration of their symptoms. Studies of how recovery sleep may counteract the deleterious effects night shifts are warranted. Changes in pain sensitivity after night shift work are of clinically relevant effect sizes, and may be important endpoints for studies comparing the physiological effects of different shift work schedules.